Журнал высшей нервной деятельности им. И.П. Павлова, 2022, T. 72, № 6, стр. 862-879
Половые различия у крыс в вальпроатной модели аутизма: нарушение социального поведения и изменение экспрессии гена Drd1 в различных структурах мозга
И. И. Семина 1, 2, *, Е. В. Валеева 1, 3, Д. О. Никитин 2, А. З. Байчурина 2, А. В. Никитина 2, Е. В. Шиловская 2, О. А. Кравцова 3
1 Центральная научно-исследовательская лаборатория, Федеральное государственное бюджетное образовательное учреждение высшего образования “Казанский государственный медицинский университет”
Министерства здравоохранения Российской Федерации
Казань, Россия
2 Кафедра фармакологии, Федеральное государственное бюджетное образовательное учреждение
высшего образования “Казанский государственный медицинский университет”
Министерства здравоохранения Российской Федерации
Казань, Россия
3 Кафедра биохимии, биотехнологии и фармакологии, Федеральное государственное автономное образовательное учреждение высшего образования “Казанский (Приволжский) федеральный университет”
Казань, Россия
* E-mail: seminai@mail.ru
Поступила в редакцию 25.10.2021
После доработки 20.02.2022
Принята к публикации 26.04.2022
- EDN: GNGUFG
- DOI: 10.31857/S0044467722060089
Аннотация
В настоящей статье с использованием экспериментальной модели аутизма, созданной путем пренатального введения вальпроевой кислоты, представлен сравнительный анализ поведенческих нарушений у самок и самцов крыс, а также показателей относительного уровня экспрессии гена дофаминовых рецепторов Drd1 в структурах головного мозга, играющих значимую роль в нарушении социального поведения и развитии тревожности: в префронтальной коре, миндалине, мозжечке и гиппокампе. Показано, что состояние тревожности в вальпроатной модели аутизма развивается только у самцов, а нарушение социального поведения характерно для крыс обоего пола, хотя характер этих нарушений у самок и самцов различается: в методе “Трехкамерный социальный тест” самцы предпочитали больше времени находиться в отсеке со знакомым животным, а самки – значительно меньше с новой, незнакомой крысой, по сравнению с контрольными животными. Обнаружены половые различия в относительном уровне экспрессии гена Drd1 в префронтальной коре, миндалине и мозжечке: у самок относительный уровень экспрессии Drd1 повышен в префронтальной коре и миндалине, тогда как у самцов, напротив, отмечено существенное его снижение в мозжечке как по отношению к самцам контрольной группы, так и по отношению к самкам. Анализ полученных результатов свидетельствует о целесообразности рассматривать изменения относительных уровней экспрессии Drd1 одновременно во всех исследуемых областях, оценивая общие измененные профили этого гена, которые могут лежать в основе половых различий как в коммуникативных нарушениях, так и в других возможных изменениях поведения в вальпроатной модели аутизма у крыс.
Расстройства аутистического спектра (РАС) – это патология психического развития, характеризующаяся ранними нарушениями социальной коммуникации и социального взаимодействия (DeFilippis, Wagner, 2016; Chang et al., 2017). РАС включают в себя весьма разнородный набор расстройств (Willsey, State 2015; Cho et al., 2017), что представляет серьезную проблему для диагностики и лечения. В настоящее время проводится большое количество исследований для понимания молекулярных механизмов этих нарушений, выявления потенциальных лекарственных мишеней и разработки подходов к коррекции поведения при аутистических расстройствах (Kennedy, Adolphs, 2012; Meyer-Lindenberg, Tost, 2012; Torre-Ubieta, 2016). Несмотря на то, что этиопатогенез РАС является сложным и многофакторным, включающим влияние генетических особенностей и половой принадлежности, было выявлено несколько патогенетических звеньев, лежащих в основе изменений социального поведения при этих расстройствах (Parletta et al., 2016; Parker et al., 2017; Horder et al., 2018; Toczylowska et al., 2020), при этом существенное значение имеет дисфункция нейромедиаторных систем и нарушение их взаимодействия (Freitag, 2010; Zieminska et al., 2018; Семина и соавт., 2019; Toczylowska et al., 2020).
В значительной мере в регулирование социального поведения вовлечена дофаминергическая система (Reguilon et al., 2017; Cao et al., 2010; Francis et al., 2015). В нашем исследовании мы сделали акцент на анализе экспрессии гена дофаминовых D1-рецепторов, которые играют значимую роль в нарушении социального поведения: чрезмерное повышение или снижение их функции в определенных структурах мозга у животных приводит к дефициту социального взаимодействия (Liu, 2017; Lee, 2017; Tickerhoof et al., 2020). D1-рецепторы вовлечены также в нарушение коммуникативного поведения у людей с РАС. В частности, было показано, что аллель С полиморфизма rs265981, аллель A полиморфизма rs4532 и аллель T полиморфизма rs686 гена DRD1 ассоциируются с РАС (Azzam et al., 2018). Имеются сведения о значении этих рецепторов в социальном поведении и у животных: мутации в гене Drd1 крыс приводят к значительным нарушениям в различных компонентах социального поведения (Homberg et al., 2016).
Наряду с доказанностью участия D1-рецепторов в социальном поведении не совсем понятна их роль в механизме нарушения социального поведения с учетом половых различий. Некоторые исследователи считают, что вовлечение D1-рецепторов в патогенез поведенческих нарушений у крыс отмечается только у самцов (Oguchi-Katayama et al., 2013), в то же время показано, что введение агонистов D1-рецепторов в прилежащее ядро мышей приводит к усилению социального взаимодействия только у самок, но не у самцов (Campi et al., 2014); о половых различиях в социальном поведении и молекулярных путях, которые регулируются рецепторами D1 у степных полевок, сообщается и в работе Tickerhoof et al., 2020.
Половые различия характерны при РАС у людей (Tillmann et al., 2018; Charman et al., 2017; Beggiato et al., 2017): аутизм в большей степени распространен у мальчиков, но сопровождается более глубокими деструктивными синдромами у девочек (Lai et al., 2015; Cho et al., 2017; Ratto et al., 2018).
Половые различия при нарушении социального поведения отмечены и при моделировании аутизма у крыс путем пренатального введения вальпроевой кислоты (Schneider et al., 2008; Nicolini, Fahnestock, 2017). Считается, что пренатальное введение вальпроевой кислоты вызывает более обширные поведенческие и молекулярные изменения у самцов, поэтому на них и сосредоточено большинство исследований. В результате отсутствует четкое понимание механизма половых различий в поведении самцов и самок, а также в молекулярных мишенях, лежащих в основе нарушенного поведения при аутизме (Kessler et al., 2016; Nicolini, Fahnestock, 2017; Lai et al., 2015; Cho et al., 2017; Ratto et al., 2018).
Учитывая совокупность этих сведений, целью нашей работы стало проведение сравнительного анализа нарушений в социальном поведении и уровней экспрессии гена Drd1 в разных отделах мозга у самцов и самок крыс в вальпроатной модели аутизма (ВМА) для получения новых сведений о механизмах изменения поведения с учетом половых различий.
МЕТОДИКА
Лабораторные животные были получены из питомника Филиал “Столбовая” Федерального государственного бюджетного учреждения науки “Научный центр биомедицинских технологий Федерального медико-биологического агентства”. До начала экспериментов все животные содержались в стандартных условиях вивария с естественным световым режимом на полнорационной сбалансированной диете (ГОСТ Р 50258-92) с соблюдением Международных рекомендаций Европейской конвенции по защите позвоночных животных, используемых при экспериментальных исследованиях (1997), а также правил “Правила надлежащей лабораторной практики”, утвержденных приказом Министерства здравоохранения РФ № 199н от 01.04.2016. Все исследования были одобрены локальным этическим комитетом ФГБОУ ВО Казанского ГМУ Минздрава России.
Дизайн эксперимента представлен на рис. 1.
Для моделирования аутизма у крыс была использована вальпроатная модель (Schneider et al., 2008). Вальпроевую кислоту (Конвулекс, G.L.PHARMA, GmbH, Австрия) вводили самкам крыс линии Wistar подкожно в дозе 500 мг/кг на 13-й день беременности, так как именно этот период является критическим для эмбрионального развития и на него приходится пик нейрогенеза и экспрессии генов в нейромедиаторных системах. Беременным самкам контрольной группы на том же сроке в том же объеме вводили подкожно физиологический раствор. Эксперименты проведены на потомстве этих крыс: 20 самцах, 20 самках из 8 пометов с пренатальным введением вальпроевой кислоты и 20 самцах, 20 самках из 7 пометов контрольных крыс.
После отнятия от матерей в возрасте примерно 35 дней животных рассаживали с учетом пола в отдельные клетки, не более 6 крыс в каждой клетке. Крыс из одного помета содержали, как правило, всех вместе в одной клетке. Таким образом, первоначально в каждой клетке находились особи примерно из двух пометов. За неделю до начала экспериментов формировали группы животных (по 10 крыс в каждой группе), в которые включали по 1–2 крысы из каждого помета. Эксперименты начинали по достижении крысами 90 ± 3 дней.
Были проведены 2 серии экспериментов: в первой серии исследовали изменения в дофаминергической системе путем оценки выраженности каталептогенного действия, вызванного введением нейролептика галоперидола. Во второй серии (эти эксперименты проводились на других группах животных, которые не использовались в опытах с галоперидоловой каталепсией) осуществлялась оценка поведенческих характеристик и уровень экспрессии гена Drd 1 в отделах головного мозга (рис. 1).
Поведенческие тесты
Поведенческие тесты проводили с интервалом 6–7 дней для всех групп крыс в последовательности, соответствующей описанию полученных результатов. После тестирования каждого животного осуществляли дезодорацию поверхности установки с использованием 3%-й перекиси водорода; после каждой группы крыс установку обрабатывали 70%-м раствором этанола.
Каталепсия, вызванная введением галоперидола. Для моделирования каталепсии (Vogel, 2008) галоперидол (ООО Озон, Россия) вводили внутрибрюшинно в дозе 1 мг/кг. Для оценки выраженности каталепсии (способность животного сохранять искусственно приданную позу) мы использовали тест “поза лектора”. Передние лапы животного помещали на горизонтальную перекладину (Установка для каталепсии, НПО “Открытая наука”, Россия), расположенную на высоте 10 см, и регистрировали продолжительность нахождения крысы в “позе лектора” в течение 2 минут на 15-й, 30-й, 90-й и 120-й минутах после введения галоперидола. Окончанием каталепсии на каждом сроке считался момент, когда крыса убирала с перекладины 2-ю лапу. Если животное не выходило из позы “лектора” в течение 2 минут, крысу снимали с перекладины и длительность каталепсии на этом сроке принимали за 120 с.
Тестирование проводили в одно и то же время суток, с 11.00 до 17.00. Оценку каталептогенного действия галоперидола у крыс в ВМА и у контрольных животных осуществляли параллельно.
Для исследования социального поведения применяли метод “Трехкамерный социальный тест”; для оценки интереса к новому несоциальному объекту использовали “Тест распознавания нового объекта”.
Трехкамерный социальный тест (Cho et al., 2017; Семенова и соавт., 2020) является общепринятым методом оценки социального поведения у грызунов. Метод основан на том, что грызуны, как правило, предпочитают проводить больше времени с другими особями (общительность) и проявляют больший интерес к новому объекту, чем к знакомому (социальная новизна). Тест помогает выявить грызунов с дефицитом общительности и социальной новизны. В эксперименте была использована установка (НПО “Открытая наука”, Россия), которая представляет собой бокс с непрозрачными стенками 120 × 80 см с тремя отсеками, имеющими отверстия для свободного перемещения животных по боксу. Размер центрального отсека составляет 40 × 80 см, боковых отсеков – 40 × 80 см. Боковые отсеки содержат два сетчатых ограждения для новых объектов.
Исследование состояло из трех 10-минутных сессий: в первой сессии тестируемое животное помещали в центральный отсек со свободным доступом в боковые отсеки для адаптации к установке; во второй сессии в левый отсек помещали “социальный стимул” (незнакомое животное, иммобилизованное в металлической клетке), а в правый – неодушевленный объект (металлическая клетка) и осуществляли видеорегистрацию поведения; в третьей сессии в правый боковой отсек помещали новый “стимул” (незнакомая крыса), тогда как “стимул”, находящийся в левом отсеке, становился знакомым, и также осуществляли видеорегистрацию поведения. В тесте оценивали время (в секундах), проведенное объектом в правом и левом отсеках во 2-й сессии (как показатель предпочтения социального объекта несоциальному), а также время, проведенное объектом исследования в отсеках с незнакомой и знакомой крысами в 3-й сессии, как показатель предпочтения социальной новизны (увеличение времени, проведенного в отсеке с незнакомцем по сравнению со временем в отсеке с уже знакомой (по предыдущей сессии) крысой (Семенова и соавт., 2020)).
Незнакомые крысы брались из разных клеток, до тестирования никогда не находились в физическом контакте с объектом исследования. Стимулом служили крысы того же пола и возраста.
Тест распознавания нового объекта (Белозерцева и соавт., 2017). Эксперименты проводили на установке круглой формы диаметром 97 см (НПО “Открытая наука”, Россия). Тест состоял из трех сессий. В первую сессию крысу помещали в установку на 10 мин (сеанс привыкания). После привыкания животное убирали. В поле размещали два одинаковых несоциальных объекта (пластмассовые объемные фигуры синего цвета) на расстоянии 40 см друг от друга. Во второй сессии крысу снова сажали в установку с этими объектами и в течение следующих 10 мин регистрировали ее поведение (обнюхивание каждого объекта, сидение вблизи объекта на расстоянии менее 1 см), затем крысу убирали и один из объектов заменяли на новый, незнакомый – в нашем случае металлический полосатый контейнер. Тестируемую крысу вновь запускали в установку на 10 мин (3-я сессия) и регистрировали продолжительность (в секундах) контакта (обнюхивание, сидение вблизи объекта) с каждым из несоциальных объектов – новым (незнакомым) и старым (знакомым). Фиксацию результатов осуществляли с использованием системы видеотрекинга. Интервал времени между сессиями составлял 2–3 мин. Основным критерием теста являлась оценка длительности контакта крыс с незнакомым и знакомым объектами по результатам 3-й сессии.
Оценку тревожности проводили с использованием теста “Приподнятый крестообразный лабиринт” (ПКЛ). Этот тест позиционируется как один из наиболее чувствительных для исследования тревожности животного (Walf, Frye, 2007; Ковалёв и соавт., 2019). Установка (НПО “Открытая наука”, Россия) представляет собой квадратную площадку и четыре перпендикулярно расположенных “рукава”: два закрытых и два открытых. Закрытые “рукава” являются аналогом норы, а открытые – потенциально опасной областью. Размер “рукавов” для крыс составляет 50 × 14 см. Лабиринт приподнят над полом на 70 см. Тестирование проводили согласно Протоколу верификации, представленному производителем (www. openscience.ru).
Эксперименты в ПКЛ осуществляли при общем освещении с использованием светодиодных потолочных ламп. Параметры освещения в открытых “рукавах” составляли 350 люкс, в закрытых – 150 люкс.
За 24 часа до эксперимента крыс контрольных и опытных групп в домашних клетках переносили из вивария в лабораторию, где крысы оставались до начала тестирования, сидя в домашних клетках. Исследования проводили в звукоизолированной комнате с постоянным световым режимом, без доступа естественного освещения и без присутствия экспериментатора.
Продолжительность теста для каждого животного составляла 5 мин. Крысу помещали в центр лабиринта, носом к открытому “рукаву”. Фиксировали следующие показатели: время пребывания в закрытых “рукавах’, время пребывания в открытых “рукавах”. До начала тестирования в ПКЛ на 5 минут помещали крысу “нулевой” группы, что было необходимо для уравнивания условий тестирования (по запаховым меткам) между первым и последующими животными.
Фиксация поведенческих изменений осуществлялась при помощи цифровой видеосистемы с использованием компьютерной программы Ethovision ХТ “Noldus” (Нидерланды) с автоматическим способом анализа треков.
Оценка уровня экспрессии гена Drd1 в отделах головного мозга
Относительный уровень экспрессии исследуемого гена осуществляли с помощью TaqMan-технологии методом ПЦР в реальном времени в образцах суммарной РНК, выделенных из различных отделов головного мозга крыс (префронтальная кора (ПФК), мозжечок, миндалина и гиппокамп).
Через 24 часа после окончания тестирования крыс в ВМА и контрольных животных последовательно декапитировали с помощью гильотины (НПО “Открытая наука”, Россия) в том же порядке, в котором проводили поведенческое тестирование, вскрывали череп, извлекали головной мозг для последующего отделения ПФК, гиппокампа, мозжечка и левой миндалины. Выделение структур мозга проводили на холоде с использованием пластины с хладоагентом. Отдельный образец мозга каждой крысы помещали в охлажденные пробирки Eppendorf и немедленно замораживали при –80°С. На другой день после забора всех структур у каждой крысы выделяли суммарную мРНК, используя реагент ExtractRNA (BC032, Евроген, Россия) согласно инструкции производителя. Качество и концентрацию выделенной тотальной РНК оценивали с помощью спектрофотометра NanoDropLite (ThermoFisher, США). Среднее количество выделенной матричной РНК по всем структурам составляло 822 ± 145 нг/мкл. Для постановки реакции обратной транскрипции использовали набор реактивов для получения кДНК MMLV RT kit (SK021, Евроген, Россия) и 500 нг общей РНК. Далее с полученными образцами кДНК проводили количественную ПЦР в двух повторностях на амплификаторе CFX96Touch™ Real-Time PCR Detection System (BioRad, США), используя коммерческие наборы TagMan для гена Drd1 (Cat. 4331182) и референсного гена Gapdh (Cat. 4448490) (AppliedBiosystems, США), а также реакционной смеси TaqMan Fast Advanced MasterMix (Cat. 4444965, ThermoFisher, США). Условия ПЦР в реальном времени соответствовали вложенному протоколу: 50°С – 2 мин, 95°С – 20 сек, 39 циклов: 95°С – 1 сек, 60°С – 20 сек. Детекция результата проходила на этапе отжига при 60°С по каналам FAM для гена Drd1, по каналу VIC – для гена Gapdh.
Статистический анализ результатов поведенческих тестов проводили в программе GraphPad Prism 8.0 с предварительной проверкой нормальности распределения в группах и последующим применением двухфакторного дисперсионного анализа ANOVA с использованием post hoc анализа Бонферрони, где факторами служили принадлежность к экспериментальной группе (контроль, ВМА) – фактор “группа”, и пол животного (самцы, самки) – фактор “пол”. Данные представлены как среднее значение ± стандартная ошибка среднего.
Анализ полученных результатов экспрессии гена. Для анализа относительного уровня экспрессии генов использовался метод Ливака (2–ΔΔCt) (Livak, 2001), согласно которому подсчет уровня проводился по формуле:
2–(CqDrd1опыт – CqGapdhопыт)-(CqDrd1контроль – CqGapdhконтроль). Нормальность распределения данных оценивали с помощью теста Шапиро-Уилка. Статистическую значимость различий результатов у групп животных: самцыВМА/самцы-контроль; самкиВМА/самки-контроль, а также между группами самцыВМА/самкиВМА – определяли с использованием программы GraphPad prism 8.0.1 с вычислением t-критерия Стъюдента. Фактический уровень значимости с использованием поправок Бонферрони принято было считать p ≤ 0.01.
РЕЗУЛЬТАТЫ ИССЛЕДОВАНИЙ
Результаты исследования поведения крыс в ВМА в методе “Трехкамерный социальный тест” представлены на рис. 2. Анализ показателей во 2-й сессии теста не показал изменений в предпочтении социального объекта несоциальному у самок и самцов в ВМА по сравнению с контрольными крысами (рис. 2 – I). Применение двухфакторного ANOVA при исследовании времени нахождения в отсеке с несоциальным объектом не выявило влияния фактора “пол” [F(1.36) = 1.356, p = 0.2519], а также фактора “группа” [F(1.36) = 0.0097, p = = 0.9218], хотя установлено статистически значимое взаимодействие факторов “пол” × × “группа” [F(1.36) = 5.133, p = 0.0296)]. Проведение последующего post hoc анализа не показало статистически значимых различий у крыс в ВМА по сравнению с контрольными животными (рис. 2 – I (а)) ни в группе самок (p = 0.2065), ни в группе самцов (p = 0.2685), тем не менее были выявлены статистически значимые различия между самками и самцами в ВМА: самцы в 1.6 раза (p = 0.0479) дольше находились в отсеке с несоциальным объектом.
Рис. 2.
Поведение крыс в ВМА в методике “Трехкамерный социальный тест” во 2-й сессии (I) и 3-й сессии (II). По оси абсцисс – группы животных: К – контроль, О – крысы в ВМА; по оси ординат: I (а) – время (с), проведенное объектом исследования в камере с несоциальным объектом (металлическая клетка); I (б) – время (с), проведенное объектом исследования в камере с социальным объектом (крыса-стимул в металлической клетке); II (а) – время (с), проведенное объектом исследования в камере с “незнакомой” крысой, II (б) – время (с), проведенное объектом исследования в камере со “знакомой” крысой. + – статистически значимые отличия между самками и самцами в ВМА, p < 0.05 (2-way ANOVA, post-hoc анализ по методу Бонферрони) – I (а); ✓– статистически значимое влияние фактора “группа” по времени нахождения крыс в отсеке с социальным объектом, p < 0.05 (2-way ANOVA) – I (б); * – статистически значимые различия между контрольной и опытной группами при р < 0.05 (2-way ANOVA, post-hoc анализ по методу Бонферрони). Fig. 2. The behavior of rats in the VРA in the “Three-chamber interaction” method in the 2nd session (I) and the 3rd session (II). On the abscissa axis – groups of animals: K – control, O – rats in the VРA; on the ordinate axis: I (а) – time (sec) spent by the research object in a cell with a non-social object (metal cage); I (б) – time (sec) spent by the research object in a chamber with a social object (stimulus rat in a metal cage); II (а) – the time (sec) spent by the research object in the chamber with the “unfamiliar” rat, II (б) – the time (sec) spent by the research object in the chamber with the “familiar” rat; + – statistically significant differences between females and males in the VPA, p < 0.05 (2-way ANOVA, post-hoc analysis according to the Bonferroni method) – I (а); ✓– statistically significant effect of the “group” factor on the time spent by rats in the compartment with a social object, p < 0.05 (2-way ANOVA) – I (б); * – statistically significant differences between the control and experimental groups at p < 0.05 (2-way ANOVA, post-hoc analysis by the Bonferroni method).
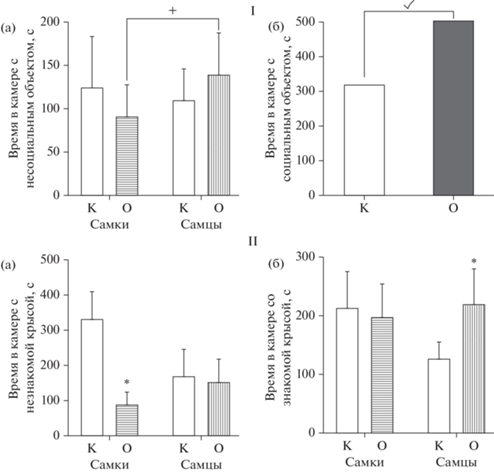
При исследовании в этой же сессии длительности нахождения крыс в отсеке с социальным объектом также не было установлено влияния фактора “пол” [F(1.36) = 0.1175, p = = 0.7338], однако выявлено влияние фактора “группа” [F(1.36) = 7.143, p = 0.0113], при этом взаимодействия факторов обнаружено не было [F(1.36) = 0.1045, p = 0.7483]. Поскольку по данному показателю было выявлено только влияние фактора “группа”, нами был проведен анализ по всей выборке животных и установлены статистически значимые различия между всеми крысами опытной и контрольной групп, т.е. крысы в ВМА, по сравнению с контрольными, проводили больше времени с социальным объектом (рис. 2 – I (б)).
Применение двухфакторного ANOVA при изучении длительности нахождения в отсеке с “незнакомой” крысой в 3-й сессии опыта не выявило влияния фактора “пол” [F(1.36) = 2.85, p = 0.102], но позволило обнаружить статистически значимое влияние фактора “группа” [F(1.36) = 18.97, p = 0.0001] и взаимодействие факторов “группа” × “пол” [F(1.36) = = 13.23, p = 0.0009]. Последующий post hoc анализ показал, что самки в ВМА в 3.6 раза (p < 0.001) меньше времени находились в отсеке с “незнакомой” крысой по сравнению с аналогичными показателями у крыс контрольной группы (рис. 2 – II (а)).
Оценка социального поведения при нахождении объекта исследования в отсеке со “знакомой” крысой в этой же сессии с использованием ANOVA не выявила влияния факторов “группа” [F(1.36) = 2.56, p = 0.1177] и “пол” [F(1.36) = 1.99, p = 0.1669], но было установлено взаимодействие между этими факторами (“группа” × “пол”) [F(1.36) = 5.40, p = = 0.0259]. Проведение post hoc анализа показало статистически значимое увеличение (в 1.5 раза, p = 0.0173) времени нахождения самцов в ВМА в отсеке со “знакомым” животным по сравнению с контрольными крысами (рис. 2 – II (б)).
Анализ параметров поведения крыс методом ANOVA в тесте “Распознавание объекта” при предъявлении им нового, незнакомого объекта (рис. 3 – I), не выявил статистически значимого влияния фактора “группа” [F(1.36) = = 0.089, p = 0.7667] и взаимодействия факторов [F(1.36) = 0.8046, p = 0.3757], однако было установлено влияние фактора “пол” [F(1.36) = 27.50, p = 0.0001]. Последующий анализ показал, что самки, вне зависимости от группы (опытной или контрольной), статистически значимо больше времени проводили с незнакомым объектом, чем самцы (рис. 3 – I (а)).
Рис. 3.
Поведение крыс в ВМА в методике “Распознавание объекта”. По оси абсцисс – группы животных: К – контроль, О – крысы в ВМА; по оси ординат: I – длительность контакта (с) исследуемой крысы со незнакомым объектом, II – длительность контакта (с) исследуемой крысы со знакомым объектом. × – статистически значимое влияние фактора “пол” по показателю времени контакта с “незнакомым объектом”, p < 0.05 (2-way ANOVA) – I (а); ✓– статистически значимое влияние фактора “группа” по показателю продолжительности контакта со знакомым объектом, p < 0.05 (2-way ANOVA) – II (а); × – статистически значимое влияние фактора “пол” по показателю продолжительности контакта со “знакомым объектом”, p < < 0.05 (2-way ANOVA) – II (б); * – статистически значимые различия между контрольной и опытной группами, р < 0.05 (2-way ANOVA, post-hoc анализ по методу Бонферрони). Fig. 3. Behavior of rats in VРA in the “Object recognition” method. On the abscissa axis – groups of animals: K – control, O – rats in the VРA; on the ordinate axis: I – duration of contact (sec) of the test rat with a unfamiliar object, II – duration of contact (sec) of a test rat with an familiar object. × – statistically significant influence of the “gender” factor in terms of the time of contact with the “unfamiliar object”, p < 0.05 (2-way ANOVA) – I (а); ✓– statistically significant influence of the “group” factor in terms of the duration of contact with a familiar object, p < 0.05 (2-way ANOVA) – II (а); × – statistically significant effect of the “gender” factor in terms of the duration of contact with a “familiar object”, p < 0.05 (2-way ANOVA) – II (б); * – statistically significant differences between the control and experimental groups, p < 0.05 (2-way ANOVA, post-hoc analysis by the Bonferroni method).
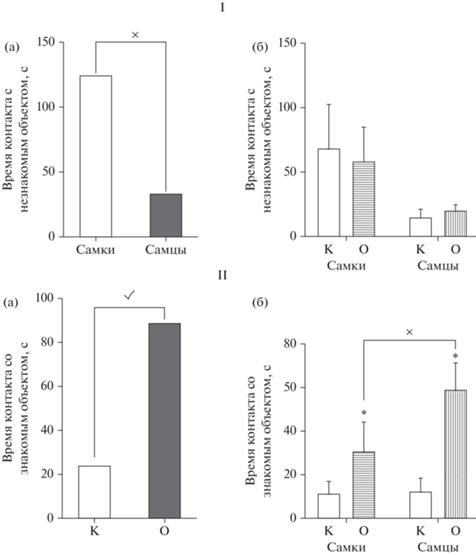
Анализ показателей времени, проведенного крысами со знакомым объектом, выявил статистически значимое влияние факторов “группа” [F(1.36) = 78.72, p < 0.0001] и “пол” [F(1.36) = 15.38, p = 0.0004], а также статистически значимое взаимодействие факторов “группа” × “пол” [F(1.36) = 13.33, p = 0.0008]. Post hoc анализ показал, что и самки, и самцы из группы с ВМА предпочитали проводить больше времени с уже знакомым им несоциальным объектом, и время нахождения с этим объектом превосходило показатели контрольной группы в 4.6 (p = 0.0015) и 2.6 (p < 0.0001) раза для самцов и самок соответственно (рис. 3 – II (б)). Кроме того, самцы опытной группы в 1.8 раза (p = 0.0248) дольше контактировали со знакомым объектом по сравнению с самками.
Учитывая, что в развитии нарушений социального поведения существенную роль играет фактор тревожности, нами было исследовано поведение крыс на установке ПКЛ (рис. 4).
Рис. 4.
Характеристики состояния тревожности самок и самцов крыс в ВМА в ПКЛ. По оси абсцисс – группы животных: К – контроль, О – крысы с ВМА; по оси ординат: I – время (с), проведенное крысой в “открытых рукавах” (ОР); II – время (с), проведенное крысой в “закрытых рукавах” (ЗР). × – статистически значимое влияние фактора “пол” на длительность нахождения крыс в ОР ПКЛ (I (а)), p < 0.05 (2-way ANOVA); ✓ – статистически значимое влияние фактора “группа” на длительность нахождения крыс в ОР ПКЛ (I (б)), p < 0.05 (2-way ANOVA); ✓– статистически значимое влияние фактора “группа” на время, проведенное в ЗР (II), p < 0.05 (2-way ANOVA). Fig. 4. Characteristics of the state of anxiety in female and male rats in VРA in EPM. On the abscissa axis - groups of animals: K – control, O – rats with VРA; on the ordinate: I – time (sec) spent by the rat in the “open arms” (OA); II – time (sec) spent by the rat in the “closed arms” (CA). × – statistically significant effect of the “gender” factor on the duration of the stay of rats in the OA EPM (I-A), p < 0.05 (2-way ANOVA); ✓ – statistically significant effect of the “group” factor on the duration of the stay of rats in the OA EPM (I- Б), p < 0.05 (2-way ANOVA); ✓ – statistically significant effect of the “group” factor on the time spent in the CA (II), p < 0.05 (2-way ANOVA).
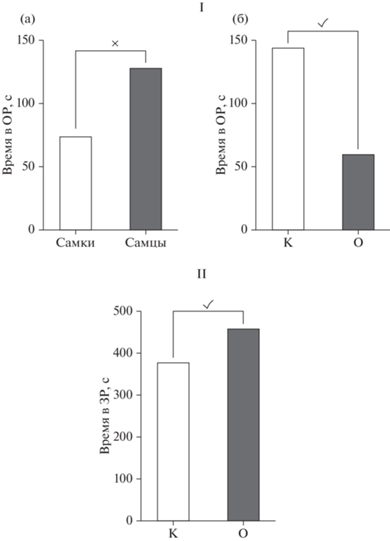
Использование двухфакторного ANOVA выявило статистически значимое влияние фактора “пол” [F(1.36) = 9.244, p = 0.0044] (рис. 4 – I (а)) и фактора “группа” [F(1.36) = = 21.20, p < 0.0001] (рис. 4 – I (б)) на длительность нахождения крыс в ОР ПКЛ. Кроме того, было выявлено значимое влияние фактора “группа” на время, проведенное в ЗР [F(1.36) = = 11.32, p = 0.0018] (рис. 4 – II), то есть все крысы в ВМА проводили больше времени в ЗР и меньше в ОР по сравнению с контрольными. При этом не было отмечено взаимодействия факторов “группа” × “пол”, как в ОР [F(1.36) = 1.64, p = 0.208], так и в ЗР [F(1.36) = = 0.7515, p = 0.3918], а также влияния фактора “пол” на время, проведенное крысами в ЗР.
Применение двухфакторного ANOVA при исследовании каталептогенного действия галоперидола, оцениваемого по длительности нахождения крыс в позе “лектора”, выявило статистически значимые эффекты влияния факторов только на 15 и 30-й минутах наблюдения. На 15-й минуте было обнаружено влияние фактора “пол” (рис. 5 (а)) [F(1.36) = 11.01, p = 0.0021] и взаимодействие факторов “группа” × “пол” [F(1.36) = 4.947, p = 0.0325], при этом влияния фактора “группа” не отмечено. Последующее использование post hoc анализа показало, что у самок в ВМА каталепсия развивалась значительно медленнее (рис. 5 (в)): длительность их нахождения в “позе лектора” была короче в 2.9 раза (p = 0.0172), чем у контрольных; следует отметить и статистически значимую разницу между самцами и самками в ВМА – у последних каталепсия развивалась в 3.15 раза медленнее, чем у самцов (р = 0.0023).
Рис. 5.
Показатели каталептогенного действия галоперидола у самок и самцов крыс с ВМА. По оси абсцисс – интервалы наблюдения (мин) и группы животных: К – контроль (галоперидол), О – крысы с ВМА + галоперидол. По оси ординат – длительность каталепсии по отношению к показателям контрольных крыс, принятым за 100%. × – статистически значимое влияние фактора “пол” на 15-й минуте наблюдения, p < 0.05 (2-way ANOVA) (а); ✓ – статистически значимое влияние фактора “группа” на 30-й минуте наблюдения, p < 0.05 (2-way ANOVA) (б); * – статистически значимые различия между контрольной и опытной группами, р < 0.05 (2-way ANOVA, post-hoc анализ по методу Бонферрони) (в). Fig. 5. Indicators of the cataleptogenic effect of haloperidol in female and male rats with VРA. On the abscissa axis – observation intervals (min) and groups of animals: К – control (haloperidol), O – rats with VPA + haloperidol; on the ordinate axis – duration of catalepsy in relation to the indicators of control rats taken as 100%. × – statistically significant influence of the “gender” factor at the 15th minute of observation, p < 0.05 (2-way ANOVA) (а); ✓ – statistically significant influence of the “group” factor at the 30th minute of observation, p < 0.05 (2-way ANOVA) (б); * – statistically significant differences between the control and experimental groups, p < 0.05 (2-way ANOVA, post-hoc Bonferroni analysis) (в).
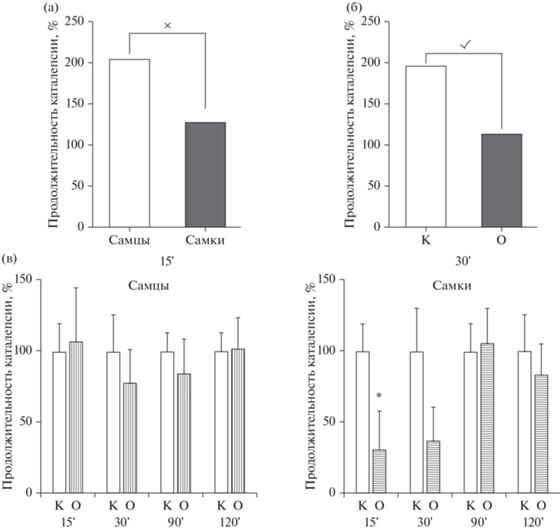
На 30-й минуте наблюдения не было обнаружено влияния фактора “пол” [F(1.36) = 1.116, p = 0.2870] и взаимодействия факторов “группа” × “пол”, но при этом отмечено влияние фактора “группа” [F(1.36) = 7.104, p = 0.0114] (рис. 5б): у всех крыс в ВМА показатели длительности каталепсии на данном сроке были меньше, чем у всех контрольных.
Таким образом, мы подтвердили, что у крыс опытной группы отмечались поведенческие нарушения, характерные для ВМА, и показали, что эти изменения выявлялись не только у самцов, но и у самок. Уменьшение каталептогенного действия галоперидола у крыс с пренатальным введением вальпроевой кислоты свидетельствует об изменениях в дофаминергической системе.
Результаты оценки относительного уровня экспрессии гена Drd1 в ПФК, миндалине, мозжечке и гиппокампе представлены на рис. 6.
Рис. 6.
Относительный уровень экспрессии гена Drd1 в структурах мозга крыс в ВМА: (а) – префронтальная кора; (б) – миндалина; (в) – мозжечок; (г) – гиппокамп. По оси абсцисс – группы животных; по оси ординат – относительный уровень экспрессии гена Drd1 (контрольные значения приняты за 1). + – тенденция к различиям между контрольной и опытной группами при 0.05 ≤ p ≤ 0.01; % – тенденция к различиям между самцами и самками p = 0.027; * – статистически значимые различия между контрольной и опытной группами при p ≤ 0.01; ** – статистически значимые различия между контрольной и опытной группами при p ≤ 0.001. # – статистически значимые различия между группами самцов и самок при p ≤ 0.01. Fig. 6. Relative level of Drd1 gene expression in the brain structures of rats with VРA: (а) – prefrontal cortex; (б) – amygdala; (в) – cerebellum; (г) – hippocampus. The abscissa shows groups of animals; ordinate – relative level of Drd1 gene expression (control values are taken as 1). + – tendency towards significance relative to the control at 0.05 ≤ p ≤ 0.01; % – tendency towards significance between males and females p = 0.027; * – the difference is significant in relation to the control at p ≤ 0.01; ** – the difference is significant in relation to the control at p ≤ 0.001; # – the difference is significant between males and females p ≤ 0.01.
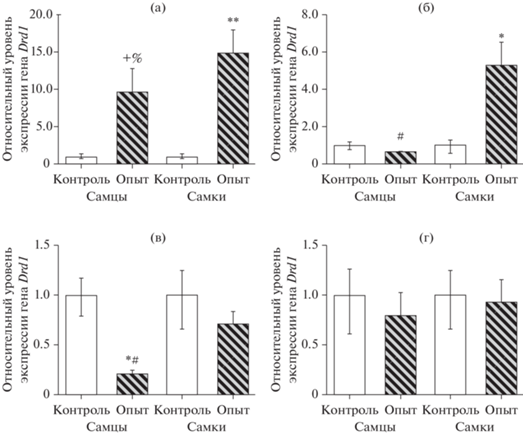
В ПФК у самок в ВМА были выявлены существенные изменения в экспрессии гена Drd1 (рис. 6а) – относительный уровень экспрессии был у них выше в 14.9 раза (p < < 0.0001); у самцов испытуемой группы отмечена тенденция к повышению (p < 0.02) – как по сравнению с показателями контрольной группы, так и по отношению к группе самок.
В миндалине (рис. 6б) относительное повышение экспрессии гена Drd1 отмечалось только у самок, и его уровень был выше в 5.2 раза (p = 0.005) по сравнению с данными контрольной группы и в 8.1 раза (p < 0.0001) по сравнению с группой самцов, у которых показатели экспрессии Drd1 не отличались от контрольных значений (p = 0.2). Иная тенденция в изменении относительного уровня экспрессии Drd1 наблюдалась в мозжечке (рис. 6 (в)): у самцов мы зафиксировали его снижение, причем весьма существенное – в 4.8 раза (p < 0.002) по сравнению с данными контрольной группы и в 3.4 раза (p < 0.0001) по сравнению с показателями у самок. В гиппокампе (рис. 6 (г)) мы не обнаружили изменений в относительном уровне экспрессии гена Drd1.
ОБСУЖДЕНИЕ РЕЗУЛЬТАТОВ
В настоящей работе мы провели сравнительный анализ поведенческих нарушений и изменений относительного уровня экспрессии гена Drd1 в структурах головного мозга, играющих значимую роль в нарушении социального поведения, у самцов и самок крыс в ВМА. Необходимость проведения данного исследования обусловлена наличием противоречивых сведений о половом диморфизме как у людей с РАС, так и на моделях на животных (Frazier et al., 2014; Ratto et al., 2017; Bölte et al., 2011; Mandy et al., 2017; Tillmann, 2018).
Изучение поведенческих нарушений в ВМА обычно проводят на самцах, исключая самок из эксперимента, считая, что у них не проявляются нарушения на этой модели (Oguchi-Katayama et al., 2013; Nicolini, Fahnestock, 2018). Некоторые исследователи не выделяют группы по половым признакам (Schneider et al., 2008; Kim et al., 2013), а другие подчеркивают, что пренатальное введение вальпроевой кислоты вызывает примерно одинаковые нарушения поведения у грызунов обоего пола с небольшими различиями (Kataoka et al., 2013; Cho et al., 2017).
Таким образом, все вышесказанное свидетельствует об отсутствии четкого представления о характере половых различий в поведенческих нарушениях в ВМА.
Как известно, основными чертами аутизма являются социальный дефицит, тревожность и стереотипность, и эти же характеристики поведения были отмечены в ВМА у грызунов (Schneider, Przewlocki, 2005; Nicolini, Fahnestock, 2018).
Мы показали, что нарушение социального поведения у крыс в ВМА характерно и для самцов, и для самок, но с некоторыми различиями. При анализе поведенческих изменений в методе “Трехкамерный социальный тест” во 2-й сессии, где оценивали предпочтение социального объекта несоциальному, нам не удалось выявить нарушений поведения, характерных для аутизма у крыс опытной группы в целом, но было отмечено, что самцы в ВМА предпочитали больше времени, чем самки, находиться в отсеке с несоциальным объектом.
Анализ социального поведения в 3-й сессии выявил, что самцы предпочитали больше времени находиться в отсеке со знакомой крысой, а самки – значительно меньше с незнакомой, то есть поведение и самок и самцов характеризовалось снижением предпочтения социальной новизны. В норме у животных при появлении нового (“незнакомого”) социального объекта приоритетом является необходимость его изучения с одновременным снижением интереса к уже “знакомому” животному. Следует отметить, что именно в области социального поведения были обнаружены половые различия и у детей с аутизмом (Centers for Disease Control and Prevention, 2014; Frazier et al., 2014; Lai et al., 2015).
В другом поведенческом тесте – “Распознавание нового объекта” – у крыс в ВМА были отмечены изменения поведения, характерные для аутизма: они предпочитали больше времени контактировать с уже знакомым им объектом, не проявляя, в отличие от контрольных крыс, особого интереса к незнакомому. Следует отметить, что у самцов длительность контакта со знакомым предметом была больше, чем у самок. Таким образом, результаты этого теста, аналогично предыдущему, подтвердили снижение показателя предпочтения новизны и у самок, и у самцов в ВМА.
Один из факторов, который существенно влияет на социальное поведение, – это тревожность, которая, в свою очередь, может оказывать влияние на инициацию и интенсивность социальных взаимодействий: чем выше тревожность, тем в большей степени будут нарушения в социальном поведении (Neuhaus et al., 2010; Blackford, Pine, 2012; Carlisi, Robinson, 2018). На усиление тревожности крыс в ВМА указывает их поведение в ПКЛ: сокращение времени нахождения в ОР и увеличение продолжительности пребывания в ЗР. Такие показатели в поведении являются типичными для крыс в ВМА (Schneider et al., 2008), но иногда, на основании результатов развития тревожности только у самцов, самки исключаются из дальнейших экспериментов (Oguchi-Katayama et al., 2013).
Анализируя результаты каталептогенного действия галоперидола, мы выявили, что у самок в ВМА каталепсия развивалась медленнее, чем у самцов. Данный эффект демонстрирует некоторые половые различия в характере изменений в дофаминергической системе. Известно, что состояние каталепсии обусловлено блокадой дофаминовых D1- и D2-рецепторов нигростриатной области (Sanberg, 1980), и снижение каталептогенного действия галоперидола может свидетельствовать об изменении их плотности и аффинитета (Vogel, 2008; Hellings et al., 2017). Нигростриатная область является одной из структур, которая вовлечена в поведенческие нарушения при аутизме, а D1-рецепторы этой области играют важную роль в развитии социального дефицита и повторяющегося поведения, характерных для расстройств аутистического спектра (Kim et al., 2016; Lee et al., 2018), а также модулируют функцию D2-рецепторов (Смагин и соавт., 2018).
Учитывая, что D1-рецепторы широко распространены в дофаминергической системе ЦНС и кодируются геном Drd1, мы провели анализ относительного уровня экспрессии этого гена у крыс в ВМА в структурах мозга, играющих значимую роль в развитии нарушений социального поведения.
В ПФК относительный уровень экспрессии гена Drd1 был существенно повышен у самок и имел тенденцию к повышению у самцов в ВМА, что позволило высказать предположение о возможном увеличении активности дофаминергической системы. Известно, что повышенный уровень дофамина в ПФК способствует нарушению социального поведения, развитию тревожности и зависит прежде всего от функционального состояния D1- и отчасти D2-рецепторов (Santana et al., 2009; Shinohara et al., 2017; Plavén-Sigray et al., 2017). Сопоставляя результаты поведения опытных крыс в ПКЛ с относительным уровнем экспрессии гена Drd1 в ПФК, можно отметить, что повышенная тревожность у крыс в ВМА сопровождается высоким уровнем экспрессии гена Drd1 у самок и тенденцией к повышению экспрессии этого гена у самцов.
Миндалина тоже относится к структурам мозга, в которых изменение функции D1-рецепторов может приводить к нарушению коммуникативных навыков и общения (Neuhaus et al., 2010; Homberg et al., 2016). Следует отметить, что в литературе имеются сведения о латеральности действия дофамина в миндалине (Bradbury et al., 1985), а также о нарушении межполушарной асимметрии при некоторых нервно-психических нарушениях и специфическом повышении содержания дофамина в левой миндалине (Reynolds, 1983). На данном этапе исследований мы не определяли латеральность действия дофамина в миндалине и ограничились пока только исследованием уровня экспрессии гена Drd1 у всех крыс в левой миндалине.
В наших экспериментах уровень гена Drd1 в миндалине изменялся только у самок в ВМА, причем был значимо выше, чем у самцов. Ранее некоторыми исследователями сообщалось об отсутствии изменений экспрессии гена Drd1 в миндалине у крыс-самцов в ВМА (Oguchi-Katayama et al., 2013). Возможно, D1-рецепторы миндалины, несмотря на их значимость в регуляции социального поведения, не принимают участия в развитии социальных нарушений в ВМА у крыс–самцов в половозрелом возрасте. Повышенный относительный уровень экспрессии гена Drd1 у самок в ВМА может быть связан с наличием у них половых гормонов – эстрогенов и прогестерона, которые способны изменять активность дофаминергической передачи, влияя на опосредованную ГАМК-регуляцию дофамина (McEwen, 2002; Baron-Cohen et al., 2019).
Патологические изменения в мозжечке при аутизме характеризуются его гипоплазией с потерей клеток Пуркинье и аномальной нейротрансмиссией, что также сопровождается симптомами социального и коммуникативного дефицита и подтверждается многочисленными исследованиями у человека (Rojas et al., 2006; Riva et al., 2013; D’Mello et al., 2015) и на животных (Stoodley et al., 2017; Hoche et al., 2016; Tsai et al., 2018). Что касается D1-рецепторов мозжечка, то их плотность по сравнению с миндалиной и ПФК невелика, но они выполняют значимую роль в регуляции в ПФК дофаминергической передачи, которая нарушается при аутизме (McKimm et al., 2014; D’Mello, Stoodley, 2015; Crippa et al., 2016).
Исследование относительного уровня экспрессии гена Drd1 в мозжечке показало существенное его снижение у самцов, причем разница была достоверна и по отношению к группе самок с экспериментальным аутизмом. Таким образом, мозжечок – это еще одна структура мозга, где мы можем видеть различия в экспрессии гена Drd1 между самцами и самками в модели аутизма.
Структурно-функциональные нарушения в гиппокампе также связаны с патогенезом поведенческих расстройств при РАС (Varghese et al., 2017; Toczylowska et al., 2020). Исследование относительного уровня экспрессии гена Drd1 в гиппокампе не выявило его статистически значимых различий ни у самок, ни у самцов в ВМА по сравнению с контрольными крысами.
Таким образом, в структурах головного мозга крыс в ВМА нами были выявлены выраженные изменения в экспрессии гена Drd1, со значимыми половыми различиями. Анализ полученных результатов показывает целесообразность рассмотрения относительных уровней экспрессии гена Drd1 одновременно во всех исследуемых областях, оценивая у самок и самцов общие измененные профили этого гена, которые могут вызывать дисбаланс уровней D1-рецепторов, способствуя нарушению межструктурного взаимодействия в дофаминергической системе мозга.
Пока на основании полученных данных мы не можем с уверенностью говорить о характере изменений функциональной рецепции – для этого необходимы исследования по определению экспрессии соответствующего функционального белка; требует также дополнительного подтверждения наличие половых различий в коммуникативных нарушениях у крыс в вальпроатной модели аутизма. Тем не менее нельзя отрицать тот факт, что измененные уровни экспрессии гена Drd1 у самок и самцов могут играть определенную роль в возможных изменениях поведения на этой модели.
ЗАКЛЮЧЕНИЕ
Представленные выше исследования показали, что изменения социального поведения у крыс в ВМА отмечаются как у самцов, так и у самок, но различаются рядом показателей. Кроме того, нами выявлены половые различия в профиле экспрессии гена Drd1 в префронтальной коре, миндалине и мозжечке. Полученные данные дополняют сведения о половом диморфизме в вальпроатной модели аутизма и имеют значение для понимания некоторых молекулярных механизмов, лежащих в основе нарушения поведения в ВМА. Учитывая гетерогенность аутизма, необходимо разрабатывать подходы к диагностике и лекарственной коррекции нарушений социального поведения и тревожности с учетом патогенетических различий, обусловленных половыми особенностями мозга.
Полученные результаты подтверждают необходимость расширения спектра фундаментальных и клинических исследований аутистических расстройств с учетом половых различий, поскольку до сих пор их природа окончательно не изучена.
Список литературы
Белозерцева И.В., Драволина О.А., Кривов В.О., Тур М.А., Мус Л.В., Полушин Ю.С. Послеоперационные изменения поведения крыс, получавших анестезию севофлураном. Вестн. анестезиологии и реаниматологии, 2017. 14(2): 55–62.
Ковалёв Г.И., Васильева Е.В., Салимов Р.М. Сравнение поведения мышей в тестах открытого поля, закрытого и приподнятого крестообразных лабиринтов с помощью факторного анализа. Журн. высш. нервн. деят. им. И.П.Павлова, 2019. 69(1): 123–130.
Семенова А.А., Лопатина О.Л., Салмина А.Б. Модели аутизма и методики оценки аутистически-подобного поведения у животных. Журн. высш. нервн. деят. им. И.П. Павлова, 2020. 70(2): 147–162.
Сёмина И.И., Мухарямова Л.М., Сабиров И.С., Валеева Е.В., Сафиуллина Л.Р., Никитин Д.О. Современное состояние проблемы расстройств аутистического спектра-некоторые медико-биологические и социально-гуманитарные аспекты. Каз. мед. журн., 2019. 100(6): 918–929.
Смагин Д.А., Галямина А.Г., Коваленко И.Л., Бабенко В.Н., Тамкович Н.В., Борисов С.А., Толстикова Т.Г., Кудрявцева Н.Н. Дифференциально экспрессирующиеся гены нейромедиаторных систем в дорсальном стриатуме самцов мышей с двигательными нарушениями. Журн. высш. нервн. деят. им. И.П.Павлова, 2018. 68(2): 227–249.
Azzam A.A.A., Bahgat D.M.R., Shahin R.M.H., Nasralla R.M.A. Association study between polymorphisms of dopamine transporter gene (SLC6A3), dopamine D1 receptor gene (DRD1), and autism. J. Med. Scient. Res., 2018. 1(1): 59–65.
Baron-Cohen S., Tsompanidis A., Auyeung B., Nørgaard-Pedersen B., Hougaard D.M., Abdallah M., Pohl A. Foetal oestrogens and autism. Mol. Psychiatry, 2019. 1–9.
Blackford J.U., Pine D.S. Neural substrates of childhood anxiety disorders: a review of neuroimaging findings. J. Am. Acad. Child. Adolesc. Psychiatry, 2012. 21(3): 501–525.
Bölte S., Duketis E., Poustka F., Holtmann M. Sex differences in cognitive domains and their clinical correlates in higherfunctioning autism spectrum disorders. Autism, 2011. 15(4), 497–511.
Bradbury A.J., Costall B., Domeney A.M., Naylor R.J. Laterality of dopamine function and neuroleptic action in the amygdala in the rat. Neuropharmacology, 1985. 24(12): 1163–1170. https://doi.org/10.1016/0028-3908(85)90149-2
Campi K.L., Greenberg G.D., Kapoor A., Ziegler T.E., Trainor B.C. Sex differences in effects of dopamine D1 receptors on social withdrawal. Neuropharmacology, 2014. 77: 208–216.
Cao J.L., Covington H.E., Friedman A.K., Wilkinson M.B., Walsh J.J., Cooper D.C., Han M.H. Mesolimbic dopamine neurons in the brain reward circuit mediate susceptibility to social defeat and antidepressant action. J. Neurosci., 2010. 30(49): 16453–16458.
Carlisi C.O., Robinson O.J. The role of prefrontal–subcortical circuitry in negative bias in anxiety: Translational, developmental and treatment perspectives. Brain Neurosci. Adv., 2018. 2: 1–12.
Chang Y.C., Cole T.B., Costa L.G. Behavioral phenotyping for autism spectrum disorders in mice. Curr. Protoc. Toxicol., 2017. 72(1): 11–22.
Charman T., Loth E., Tillmann J., Crawley D., Wooldridge C., Goyard D., Ahmad J., Auyeung B., Ambrosino S., Banaschewski T., Baron-Cohen S., Baumeister S., Beckmann C., Bölte S., Bourgeron T., Bours C., Brammer M., Brandeis D., Brogna C., de Bruijn Y., Chakrabarti B., Cornelissen I., Dell’ Acqua F., Dumas G., Durston S., Ecker C., Faulkner J., Frouin V., Garcés P., Ham L., Hayward H., Hipp J., Holt R. J., Isaksson J., Johnson M.H., Jones E.J.H., Kundu P., Lai M.-C., D’ardhuy X.L., Lombardo M.V., Lythgoe D.J., Mandl R., Mason L., Meyer-Lindenberg A., Moessnang C., Mueller N., O’Dwyer L., Oldehinkel M., Oranje B., Pandina G., Persico A.M., Ruggeri B., Ruigrok A.N. V., Sabet J., Sacco R., Cáceres A.S.J., Simonoff E., Toro R., Tost H., Waldman J., Williams S.C.R., Zwiers M.P., Spooren W., Murphy D.G.M., Buitelaar J.K. The EU-AIMS Longitudinal European Autism Project (LEAP): Clinical characterisation. Mol. Autism, 2017. 8(1): 27.
Cho H., Kim C.H., Knight E.Q., Oh H.W., Park B., Kim D.G., Park H.J. Changes in brain metabolic connectivity underlie autistic-like social deficits in a rat model of autism spectrum disorder. Sci. Rep., 2017. 7(1): 1–16. https://doi.org/10.1038/s41598-017-13642-3
Crippa A., Del Vecchio G., Busti Ceccarelli S., Nobile M., Arrigoni F., Brambilla P. Cortico-cerebellar connectivity in autism spectrum disorder: what do we know so far? Front. Psychiatry, 2016. 7:20.
D’Mello A.M., Stoodley C.J. Cerebro-cerebellar circuits in autism spectrum disorder. Front. Neurosci., 2015. 9. https://doi.org/10.3389/fnins.2015.00408
D’Mello A.M., Crocetti D., Mostofsky S.H., Stoodley C.J. Cerebellar gray matter and lobular volumes correlate with core autism symptoms. Neuroimage Clin., 2015. 7: 631–639. https://doi.org/10.1016/j.nicl.2015.02.007
DeFilippis M., Wagner K.D. Treatment of autism spectrum disorder in children and adolescents. Psychopharmacol. Bull., 2016. 46(2): 18–41.
Francis T.C., Chandra R., Friend D.M., Finkel E., Dayrit G., Miranda J., Lobo M.K. Nucleus accumbens medium spiny neuron subtypes mediate depression-related outcomes to social defeat stress. Biol. Psychiatry, 2015. 77(3): 212–222.
Frazier T.W., Georgiades S., Bishop S.L., Hardan A.Y. Behavioral and cognitive characteristics of females and males with autism in the Simons Simplex Collection. J. Am. Acad. Child. Adolesc. Psychiatry, 2014. 53(3): 329–340.
Freitag C.M., Staal W., Klauck S.M., Duketis E., Waltes R. Genetics of autistic disorders: review and clinical implications. Eur. Child. Adolesc. Psychiatry, 2010. 19(3): 169–178.
Hellings J.A., Arnold L.E., Han J.C. Dopamine antagonists for treatment resistance in autism spectrum disorders: review and focus on BDNF stimulators loxapine and amitriptyline. Expert. Opin. Pharmacother., 2017. 18(6): 581–588.
Hoche F., Guell X., Sherman J.C., Vangel M.G., Schmahmann J.D. Cerebellar Contribution to Social Cognition. The Cerebellum, 2016. 15(6): 732–743. https://doi.org/10.1007/s12311-015-0746-9
Homberg J.R., Olivier J.D., VandenBroeke M., Youn J., Ellenbroe A.K., Karel P., Langedijk J., Shan L., van Boxte R., Ooms S., Balemans M., Muller M., Vriend G., Cools A.R., Cuppen E., Ellenbroek B.A. The role of the dopamine D1 receptor in social cognition: studies using a novel genetic rat model. Dis. Model. Mech., 2016. 9(10): 1147–1158.
Horder J., Petrinovic M.M., Mendez M.A., Bruns A., Takumi T., Spooren W., Barker J.G., Künnecke B., Murphy D.G. Glutamate and GABA in autism spectrum disorder—a translational magnetic resonance spectroscopy study in man and rodent models. Transl. Psychiatry, 2018. 8(1): 106. https://doi.org/10.1038/s41398-018-0155-1
Kataoka S., Takuma K., Hara, Y., Maeda Y., Ago Y., Matsuda T. Autism-like behaviours with transient histone hyperacetylation in mice treated prenatally with valproic acid. Int. J. Neuropsychopharmacol., 2013. 16(01): 91–103. https://doi.org/10.1017/s1461145711001714
Kennedy D.P., Adolphs R. The social brain in psychiatric and neurological disorders. Trends Cogn. Sci., 2012. 16(11): 559–572.
Kessler K., Seymour R.A., Rippon G. Brain oscillations and connectivity in autism spectrum disorders (ASD): new approaches to methodology, measurement and modelling. Neurosci. Biobehav. Rev., 2016. 71: 601–620.
Kim H., Lim C.-S., Kaang B.-K. Neuronal mechanisms and circuits underlying repetitive behaviors in mouse models of autism spectrum disorder. Behav. Brain Funct., 2016. 12(1). https://doi.org/10.1186/s12993-016-0087-y
Kim K.C., Kim P., Go H.S., Choi C.S., Park J.H., Kim H.J., Ryu J.H. Male specific alteration in excitatory postsynaptic development and social interaction in prenatal valproic acid exposure model of autism spectrum disorder. J. Neurochem., 2013. 124(6): 832–843.
Lai M.C., Lombardo M.V., Auyeung B., Chakrabarti B., Baron-Cohen S. Sex/gender differences and autism: setting the scene for future research. J. Am. Acad. Child. Adolesc. Psychiatry, 2015. 54(1): 11–24.
Lee Y., Kim H., Kim J.E., Park J.Y., Choi J., Lee J.E., Han P.L. Excessive D1 dopamine receptor activation in the dorsal striatum promotes autistic-like behaviors. Mol. Neurobiol., 2018. 55(7): 5658–5671.
Liu Q., Shi J., Lin R., Wen T. Dopamine and dopamine receptor D1 associated with decreased social interaction. Behav. Brain Res., 2017. 324: 51–57.
Livak K.J., Schmittgen T.D. Analysis of relative gene expression data using real-time quantitative PCR and the 2− ΔΔCT method. Methods, 2001. 25(4): 402–408.
Mandy W., Lai M.C. Towards sex-and gender-informed autism research. Autism, 2017. 21(6): 643–645.
McEwen B. Estrogen actions throughout the brain. Rec. Prog. Hrm. Res., 2002. 57: 357–384.
McKimm E., Corkill B., Goldowitz D., Albritton L. M., Homayouni R., Blaha C.D., Mittleman G. Glutamate Dysfunction Associated with Developmental Cerebellar Damage: Relevance to Autism Spectrum Disorders. The Cerebellum, 2014. 13(3): 346–353. https://doi.org/10.1007/s12311-013-0541-4
Meyer-Lindenberg A., Tost H. Neural mechanisms of social risk for psychiatric disorders. Nat. Neurosci., 2012. 15(5): 663–668.
Neuhaus E., Beauchaine T.P., Bernier R. Neurobiological correlates of social functioning in autism. Clin. Psychol. Rev., 2010. 30(6): 733–748.
Nicolini C., Fahnestock M. The valproic acid-induced rodent model of autism. Exp. Neurol., 2018. 299: 217–227.
Oguchi-Katayama A., Monma A., Sekino Y., Moriguchi T., Sato K. Comparative gene expression analysis of the amygdala in autistic rat models produced by pre-and post-natal exposures to valproic acid. J. Toxicol. Sci., 2013. 38(3): 391–402.
Parker K.J., Oztan O., Libove R.A., Sumiyoshi R.D., Jackson L.P., Karhson D.S., Carson D.S. Intranasal oxytocin treatment for social deficits and biomarkers of response in children with autism. Proc. Natl. Acad. Sci., 2017. 114(30): 8119–8124.
Parletta N., Niyonsenga T., Duff J. Omega-3 and Omega-6 Polyunsaturated Fatty Acid Levels and Correlations with Symptoms in Children with Attention Deficit Hyperactivity Disorder, Autistic Spectrum Disorder and Typically Developing Controls. PLoS One, 2016. 11(5): e0156432. https://doi.org/10.1371/journal.pone.0156432
Plavén-Sigray P., Hedman E., Victorsson P., Matheson G.J., Forsberg A., Djurfeldt D.R., Rück C., Halldin C., Lindefors N., Cervenka S. Extrastriatal dopamine D2-receptor availability in social anxiety disorder. Eur. Neuropsychopharmacol, 2017. 27(5): 462–469.
Ratto A.B., Kenworthy L., Yerys B.E., Bascom J., Wieckowski A.T., White S.W., Wallace G.L., Pugliese C., Schultz R.T., Ollendick T.H., Scarpa A., Seese S. What about the girls? Sex-based differences in autistic traits and adaptive skills. J. Autism Dev. Disord., 2018. 48(5): 1698–1711. https://doi.org/ 3-017-3413-9.https://doi.org/10.1007/ s1080
Reguilón M.D., Montagud-Romero S., Ferrer-Pérez C., Roger-Sánchez C., Aguilar M.A., Miñarro J., Rodríguez-Arias M. Dopamine D2 receptors mediate the increase in reinstatement of the conditioned rewarding effects of cocaine induced by acute social defeat. Eur. J. Pharmacol., 2017. 799: 48–57.
Reynolds G.P. Increased concentrations and lateral asymmetry of amygdala dopamine in schizophrenia. Nature, 1983. 305(5934): 527–529. https://doi.org/10.1038/305527a0
Riva D., Annunziata S., Contarino V., Erbetta A., Aquino D., Bulgheroni S. Gray Matter Reduction in the Vermis and CRUS-II Is Associated with Social and Interaction Deficits in Low-Functioning Children with Autistic Spectrum Disorders: a VBM-DARTEL Study. The Cerebellum, 2013. 12(5): 676–685. https://doi.org/10.1007/s12311-013-0469-8
Rojas D.C., Peterson E., Winterrowd E., Reite M.L., Rogers S.J., Tregellas J.R. Regional gray matter volumetric changes in autism associated with social and repetitive behavior symptoms. BMC Psychiatry, 2006. 6(1): 56. https://doi.org/10.1186/1471-244x-6-56
Sanberg P.R. Haloperidol-induced catalepsy is mediated by postsynaptic dopamine receptors. Nature, 1980. 284(3): 472–473.
Santana N., Mengod G., Artigas F. Quantitative analysis of the expression of dopamine D1 and D2 receptors in pyramidal and GABAergic neurons of the rat prefrontal cortex. Cereb. Cortex, 2009. 19: 849–860.
Schneider T., Przewłocki R. Behavioral alterations in rats prenatally exposed to valproic acid: animal model of autism. Neuropsychopharmacology, 2005. 30(1): 80–89.
Schneider T., Roman A., Basta-Kaim A., Kubera M., Budziszewska B., Schneider K., Przewłocki R. Gender-specific behavioral and immunological alterations in an animal model of autism induced by prenatal exposure to valproic acid. Psychoneuroendocrinology, 2008. 33(6): 728–740.
Shinohara R., Taniguchi M., Ehrlich A.T., Yokogawa K., Deguchi Y., Cherasse Y., Lazarus M., Urade Y., Ogawa A., Kitaoka S., Sawa A., Narumiya S., Furuyashiki T. Dopamine D1 receptor subtype mediates acute stressinduced dendritic growth in excitatory neurons of the medial prefrontal cortex and contributes to suppression of stress susceptibility in mice. Mol. Psychiatry, 2017. 23: 1717–1730.
Stoodley C.J., D’Mello A.M., Ellegood J., Jakkamsetti V., Liu P., Nebel M.B., Gibson M.J., Kelly E., Meng F., Cano A.C., Pascual M.J., Mostofsky S.H., Lerch J.P., Tsai P.T. Altered cerebellar connectivity in autism and cerebellar-mediated rescue of autism-related behaviors in mice. Nat. Neurosci., 2017. 20(12): 1744–1751. https://doi.org/10.1038/s41593-017-0004-1
Tickerhoof M.C., Hale L.H., Butler M.J., Smith A.S. Regulation of defeat-induced social avoidance by medial amygdala DRD1 in male and female prairie voles. Psychoneuroendocrinology, 2020. 113: 104542.
Tillmann J., Ashwood K., Absoud M., Bölte S., Bonnet-Brilhault F., Buitelaar J.K., De Bildt A. Evaluating sex and age differences in ADI-R and ADOS scores in a large European multi-site sample of individuals with autism spectrum disorder. J. Autism Dev. Disord., 2018. 48(7): 2490–2505.
Toczylowska B., Zieminska E., Senator P., Lazarewicz J.W. Hippocampal Metabolite Profiles in Two Rat Models of Autism: NMR-Based Metabolomics Studies. Mol. Neurobiol., 2020. 57: 3089–3105. https://doi.org/10.1007/s12035-020-01935-0
Torre-Ubieta L., Won H., Stein J.L., Geschwind D.H. Advancing the understanding of autism disease mechanisms through genetics. Nat. Med., 2016. 22(4): 345–361.
Tsai P.T., Rudolph S., Guo C., Ellegood J., Gibson J.M., Schaeffer S.M., Sahin M. Sensitive Periods for Cerebellar-Mediated Autistic-like Behaviors. Cell Rep., 2018. 25(2): 357–367.e4. https://doi.org/10.1016/j.celrep.2018.09.039
Varghese M., Keshav N., Jacot-Descombes S., Warda T., Wicinski B., Dickstein D.L., Harony‑Nicolas H., De Rubeis S., Drapeau E., Buxbaum J.D., Hof P.R. Autism spectrum disorder: neuropathology and animal models. Acta. Neuropathol., 2017. 134(4): 537–566. https://doi.org/10.1007/s00401-017-1736-4
Vogel H.G. Drug discovery and evaluation: pharmacological assays. Springer-Verlag, Berlin Heidelberg New York, 2008. pp. 715–774.
Walf A.A., Frye C.A. The use of the elevated plus maze as an assay of anxiety-related behavior in rodents. Nat. Protoc., 2007. 2(2): 322–328.
Willsey A.J., State M.W. Autism spectrum disorders: from genes to neurobiology. Curr. Opin. Neurobiol., 2015. 30: 92–99. https://doi.org/10.1016/j.conb.2014.10.015
Zieminska E., Toczylowska B., Diamandakis D., Hilgier W., Filipkowski R.K., Polowy R., Orzel J., Gorka M., Lazarewicz J.W. Glutamate, Glutamine and GABA Levels in Rat Brain Measured Using MRS, HPLC and NMR Methods in Study of Two Models of Autism. Front. Mol. Neurosci., 2018. 11: 418. https://doi.org/10.3389/fnmol.2018.00418
Дополнительные материалы отсутствуют.
Инструменты
Журнал высшей нервной деятельности им. И.П. Павлова