Коллоидный журнал, 2023, T. 85, № 5, стр. 682-704
Биосовместимые гидрогели на основе биоразлагаемых полиэфиров и их сополимеров
Ю. С. Фомина 1, А. С. Семкина 1, 2, Ю. Д. Загоскин 1, *, М. М. Алексанян 3, С. Н. Чвалун 1, 2, Т. Е. Григорьев 1
1 Федеральное государственное бюджетное учреждение “Национальный исследовательский центр “Курчатовский институт”
123182 Москва, пл. Академика Курчатова, 1, Россия
2 Федеральное государственное бюджетное учреждение науки Институт синтетических полимерных материалов имени Н.С. Ениколопова Российской академии наук
117393 Москва, Профсоюзная ул., 70/2, Россия
3 Федеральное государственное бюджетное научное учреждение “Российский научный центр хирургии имени академика Б.В. Петровского”, ГСП-1
119991 Москва, Абрикосовский пер., 2, Россия
* E-mail: zagos@inbox.ru
Поступила в редакцию 26.06.2023
После доработки 03.08.2023
Принята к публикации 03.08.2023
- EDN: ZAFXKE
- DOI: 10.31857/S0023291223600554
Аннотация
Гидрогели на основе полиэтиленгликоля достаточно давно зарекомендовали себя в качестве перспективных материалов для различных биомедицинских технологий. В обзоре рассмотрены системы на основе наиболее распространeнных и изученных сополимеров полиэтиленгликоля и биоразлагаемых полиэфиров лактида и гликолида. Показаны традиционные и современные подходы синтеза сополимеров и получения гидрогелей на их основе, отражены исследования структуры и свойств материалов, а также основные направления применения данных изделий на практике.
ВВЕДЕНИЕ
Гидрогелевые материалы достаточно давно нашли свое применение во многих областях науки и технологии. За счет способности удерживать воду такие системы могут применяться в сельском хозяйстве для сохранения влаги в почве [1–4], а также в различных биомедицинских областях: от контактных линз для коррекции зрения [5, 6] до раневых покрытий [7–10] и основы тканеинженерных конструкций [11–13]. Гидрогели, как правило, представляют собой набухшие в водной среде полимерные сетки. В качестве основы гидрогелей могут выступать как полимеры природного происхождения – коллаген [14], альгинат [15], каррагинан [16], хитозан [17], так и синтетического – полиэтиленгликоль (ПЭГ) [18], поливиниловый спирт [19]. Особый интерес с точки зрения применения гидрогелей в биомедицине представляют материалы на основе ПЭГ. Данный полиэфир биосовместимый, небиоразлагаемый, хорошо удерживает воду и легко выводится из организма при относительно низких молекулярных массах. В то же время его сополимеризация с биоразлагаемыми гидрофобными полиэфирами позволяет создавать полимерные сетки с контролируемой структурой и свойствами.
В данном обзоре рассмотрены две наиболее распространeнные и изученные системы сополимеров ПЭГ и биоразлагаемых полиэфиров лактида (ПЛА) и гликолида. Обзор объединяет синтез сополимеров различного строения, раскрывает методы получения гидрогелей и способы исследования их структуры, а также описывает последние результаты в области применения разрабатываемых гидрогелевых материалов. Большая часть рассматриваемых работ представлена за последние 5 лет, при этом в обзор включены и ранние классические исследования для лучшего представления о структуре и свойствах материалов. Основная цель обзора заключается в систематизации знаний в выбранной области.
1. ГИДРОГЕЛИ НА ОСНОВЕ СОПОЛИМЕРОВ ПОЛИЭТИЛЕНГЛИКОЛЯ И ПОЛИЛАКТИДА
Биосовместимая и биоразлагаемая система блок-сополимеров полиэтиленгликоль-полилактид (ПЭГ-ПЛА) является одной из возможных матриц для создания гидрогелевых материалов вследствие агрегации гидрофобных блоков ПЛА в водной среде с образованием узлов сетки гидрогеля и их структурообразования за счет проходных цепей ПЭГ. Гидрофильно-гидрофобный баланс высокомолекулярных соединений в составе гидрогелей будет оказывать существенное влияние на структуру и физико-механические свойства материалов.
Сополимеры, способные к образованию гидрогелей, могут обладать различной молекулярной структурой: блочной (диблок- (АВ), триблок- (АВА или ВАВ) и мультиблок-сополимеры) или привитой, где А и В − гидрофобный и гидрофильный блоки соответственно [20–22].
1.1. Синтез блок-сополимеров
Синтез блочных амфифильных сополимеров ПЛА и ПЭГ наиболее часто осуществляется методом полимеризации с раскрытием цикла мономера лактида. Главным преимуществом данной методики является отсутствие воды в продуктах, в отличие от реакций поликонденсации, что позволяет получать соединения с более высокой молекулярной массой. Данный синтез может быть проведен как в растворе, так и в расплаве в присутствии различных катализаторов. В частности, сообщается об исследованияx по применению таких катализаторов, как Sb2O3 [23], GeO2 [23], SnO2 [23], SnCl2 [24], изопропилат алюминия [25, 26], алкоголяты редкоземельных элементов [27, 28], Zn [29], CaH2 [29] и др. Также провeдены исследования по сополимеризации с раскрытием цикла без использования катализатора, однако синтез при температуре 140oC в течение 4–8 сут показал недостаточно высокие степени конверсии [30].
Одной из наиболее устоявшихся методик является синтез блок-сополимеров ПЭГ-ПЛА на катализаторе 2-этилгексаноат олова (II) (Sn(Oct)2) в растворе (растворитель – толуол) [31–34] или расплаве [35–39] (рис. 1).
Данная методика актуальна и в настоящее время [39]. Соединения олова показали наибольшую эффективность, однако их существенным недостатком является относительно высокая токсичность [40]. В связи с этим Sn(Oct)2 приобрел широкую распространенность, т.к. органические соли олова обладают более низкой токсичностью по сравнению с другими его соединениями [41].Еще одним известным подходом является полимеризация с раскрытием цикла лактида в растворе (растворитель − дихлорметан) на катализаторе 1,8-диазабицикло[5.4.0]ундец-7-ен (DBU). Основными преимуществами данного подхода являются неметаллическая природа катализатора, более мягкие условия проведения процесса, а также более простые методы очистки синтезированных продуктов от остаточного катализатора [42–44].
Помимо устоявшихся известных подходов к синтезу существует направление исследования по разработке альтернативных способов получения блок-сополимеров, например, с использованием принципов “зеленой” химии. В работе [45] Mhiri и соавт. предложили химическую сшивку по реакции Дильса–Альдера функционализированных ПЛА и ПЭГ (рис. 2). ПЭГ модифицировали 3-(3-фурфурилмеркаптанпропокси)-пропан-1,2-диолом и 4,4'-метилен-бис-циклогексилизоциантом (ПЭГ-F60). ПЛА был функионализирован по реакции с глицерином, 4,4'-метилен-бис-циклогексилизоциантом с последующей конденсацией концевого изоцианата со спиртовой группой N‑гидроксиметилмалеимида (ПЛА-Tri M). Далее по реакции Дильса–Альдера получали сополимер при температуре 90°C без использования катализаторов и растворителей.
Помимо непосредственно синтеза блок-сополимеров ПЭГ-ПЛА, актуальной задачей также является их модификация, которая позволяет регулировать физико-химические свойства сополимеров и, как следствие, характеристики получаемых из них материалов. В работе [46] Buwal-da и соавт. модифицировали концевые группы 8-лучевых блок-сополимеров ПЭГ-П(L)ЛА и ПЭГ-П(D)ЛА акрилатами при действии акрилоилхлорида в дихлорметане в течение 18 ч [46]. Такая реакция позволяет впоследствии получать на основе звездообразных сополимеров методом фотополимеризации стабильные и достаточно прочные гидрогели. Pertici и соавт. синтезировали тройные блок-сополимеры П(D,L)ЛА-ПЭГ-П(D,L)ЛА и в несколько стадий модифицировали их с помощью поли-N-изопропилакриламида (PNI-PAAm) [47]. Сначала концевые группы блок-сополимера реагируют с акрилоилхлоридом в ТГФ в течение 20 ч с последующим радикальным 1,2-присоединением алкоксиамина N-(2-метилпропил)-N-(1-диэтилфосфонат-2,2-диметилпропил)-O-(2-карбоксипроп-2-ил)гидроксиламин (MAMA-SG1) в 1,4-диоксане. После чего проводят полимеризацию по концевым фрагментам N-изопропилакриламидом в 1,4-диоксане. Введение блоков PNIPAAm позволяет получать термочувствительные гидрогели на основе данных сополимеров. Способность PNIPAAm к температурно-зависимым фазовым переходам известна еще с 1960-х годов [48, 49]. В работе [50] Trinh и соавт. тройные блок-сополимеры лактида и этиленгликоля модифицировали олигосерином посредством взаимодействия П(D,L)ЛА-ПЭГ-П(D,L)ЛА с сульфонамидом серина в дициклогексилкарбоимиде (DCC) в присутствии катализатора 4-диметиламинопиридин (DMAP). Такая реакция приводит к расширению спектра свойств сополимеров и биоматериалов на их основе. Внедрение олигосериновых фрагментов позволяет получать термо- и рН-чувствительные гидрогели. Grosjean и соавт. изучали влияние функционализации 8‑лучевых звездообразных ПЭГ-П(D,L)ЛА на адгезию гидрогелевых материалов [51]. Сополимеры модифицировали акрилатами, метакрилатами и пирокатехином, что приводило к различному механизму адгезии гидрогелeй к модельным тканям (за счет физическиx взаимодействий, водородныx связeй, ковалентныx сшивoк и т.д.). В работе [38] Darge и соавт. блок-сополимеры П(D,L)ЛА-ПЭГ-П(D,L)ЛА модифицировали ангидридом янтарной кислоты для получения концевых карбоксильных групп, а также 3-карбамоил-5-метилгексановой кислотой для функционализации сополимеров аминогруппой. Смесь амино- и карбокси-концевых сополимеров образует гидрогели с фазовым переходом при температуре тела, а также заметно повышает их механическую прочность.
1.2. Получение гидрогелей
Гидрогели могут быть получены как вследствие физических взаимодействий из-за агрегации гидрофобных блоков в водной фазе, так и посредством химических сшивок в результате действия сшивающих агентов, температуры или излучения. Такие материалы могут быть стимул-чувствительными при наличии определенных групп (рН-чувствительные), различных длин блоков (термочувствительные) и т.д.
Основной методикой получения физически сшитых гидрогелей является диспергирование полимера в водной фазе при наличии или отсутствии нагревания. Введение каких-либо низкомолекулярных веществ в гидрогель возможно проводить как на стадии самоорганизации надмолекулярной структуры, так и после.
В работе [39] Liu и соавт. получили гидрогель на основе осушенного сополимерa ПЭГ-П(L)ЛА-ПЭГ путем его диспергирования в деионизированной воде при нагревании до 60°C с последующим охлаждением на ледяной бане. Затем в гидрогель импрегнировали два лекарственных препарата (паклитаксел в ПЛА наночастицах и цисплатин). Таким образом был получен термочувствительный гидрогель, золь–гель переходы которого были изучены классическим способом переворачивания пробирки. Похожие методики прослеживаются в ряде исследований [36, 38, 42, 44, 53]. В другой работе сополимеры П(D,L)ЛА-ПЭГ-П(D,L)ЛА диспергировали в фосфатном буферном растворе (рН 8.0) и смешивали с раствором циклофосфамида в аналогичном буфере [52]. Похожий метод описан также в работе Pertici и соавт. [47].
Принципиально другим подходом к получению гидрогелей является химическое сшивание посредством образования ковалентных связей. В работе [54] Zhao и соавт. использовали 4-лучевые ПЭГ, модифицированные аминогруппой (тетра-ПЭГ-AM) и сукцинимидилсукцинатом (тетра-ПЭГ-SS). Сшивание было реализовано в три этапа, сначала были проведены по отдельности реакции избытка тетра-ПЭГ-AM с недостатком тетра-ПЭГ-SS и наоборот. На последней стадии полученные полимеры смешивали, в результате чего происходило образование сильно разветвленной полимерной сети. Такой подход позволяет получать гидрогелевые материалы при достаточно низком содержании полимера. После сшивки гидрогель смешивали с мицеллами на основе двойных блок-сополимеров ПЭГ-ПЛА, нагруженных лекарственными препаратами (тимололом и латанопростом).
Также существуют научные работы, которые сочетают оба подхода: физическое и химическое сшивание сетки высокомолекулярного соединения. В работе [46] 8-лучевые сополимеры П(L)ЛА-ПЭГ и П(D)ЛА-ПЭГ были модифицированы акрилатными концевыми группами. На первой стадии смесь сополимеров диспергировали в дистиллированной воде, в результате чего происходило формирование стереокомплексного гидрогеля за счет проходных цепей ПЭГ. На следующем этапе физические гидрогели подвергались сшивке под действием УФ-излучения в присутствии фотоинициатора Ingacure 2959. Для химически сшитых гидрогелей наблюдалось значительное улучшение механических характеристик.
1.3. Структура гидрогелей
Для изучения структуры гидрогелевых материалов наиболее часто применяют методы рентгеновского и нейтронного рассеяния. В работе [55] Agrawal и соавт. изучали процессы самоорганизации ПЛА-ПЭГ-ПЛА в зависимости от длины и кристалличности ПЛА-блока методом малоуглового рассеяния нейтронов (МУРН) (рис. 3). Тройные блок-сополимеры П(D,L)ЛА-ПЭГ-П(D,L)ЛА с аморфным гидрофобным блоком образовывали сферические мицеллы (радиус 10–14 нм, на рис. 3а обозначено R2) в разбавленных растворах, c увеличением концентрации сополимера наблюдали формированиe полимерной сетки за счет проходных цепей. Сополимеры с кристаллическими блокaми на основе L-лактида приводили к образованию несферических ламеллярных мицелл (радиус 11–15 нм, на рис. 3б обозначено R; ширина 8–10 нм, на рис. 3б обозначено d) в разбавленных растворах и формированию сетчатой структуры из беспорядочно ориентированных агрегатов в концентрированных растворах [55].
Рис. 3.
(а) Цветкоподобные (аморфный гидрофобный блок) и (б) ламеллярные (кристаллический гидрофобный блок) мицеллы, адаптировано с разрешения [55].
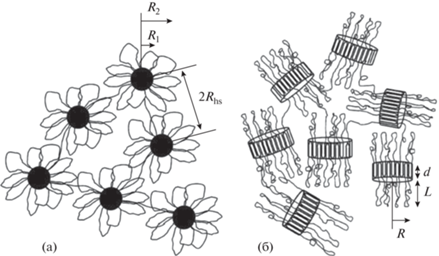
В случае сополимеров с аморфным гидрофобным блоком при увеличении концентрации мицеллы сближаются и упорядочиваются − при концентрациях выше 10% появляется корреляционный пик, который соответствует расстоянию между рассеивающими центрами в гидрогеле (рис. 4а). При одинаковой концентрации 30% сополимеры с различной длиной гидрофобного блока дают похожие кривые рассеяния, для которых наблюдается наложение практически во всем диапазоне векторoв рассеяния, что свидетельствует о схожей наноструктуре материалов (рис. 4б).
Рис. 4.
(а) Изменение спектров МУРН при увеличении концентрации сополимера П(D,L)ЛА-ПЭГ-П(D,L)ЛА со степенью полимеризации гидрофобного блока 72 (концентрация сополимера – $\square ,\,\,\bigcirc ,\,\,\vartriangle , - $); (б) Изменение спектров МУРН с увеличением длины блока ПЛА растворов сополимеров серии П(D,L)ЛА-ПЭГ-П(D,L)ЛА с рацемической смесью лактида (длина гидрофобного блока сополимера – $\square ,\,\,\bigcirc ,\,\,\vartriangle ,\,\,\diamondsuit $), адаптировано из работы [55].
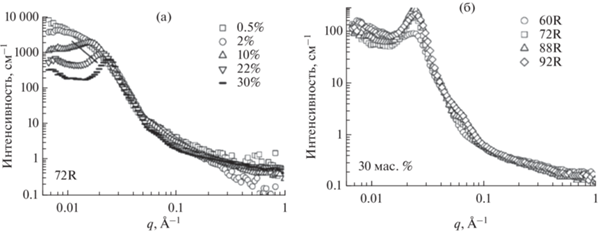
На аналогичных зависимостях для сополимеров с кристаллическим гидрофобным блоком при увеличении концентрации отсутствует корреляционный пик, хотя при концентрациях выше 22% появляется выступ. Его можно рассматривать как широкий пик, связанный с высокой полидисперсностью по размерам ламеллярных мицелл, расстояниям между ними и случайной ориентацией в пространстве (рис. 5а).
Рис. 5.
Спектры МУРН: (а) изменение спектров МУРН при увеличении концентрации сополимера П(L)ЛА-ПЭГ-П(L)ЛА со степенью полимеризации блока 72 (концентрация сополимера – $\square ,\,\,\bigcirc ,\,\,\vartriangle , - $) ; (б) изменение спектров МУРН с увеличением длины блока ПЛА растворов сополимеров серии П(L)ЛА-ПЭГ-П(L)ЛА с L-лактидом (длина гидрофобного блока сополимера – $\square ,\,\,\bigcirc ,\,\,\vartriangle ,\,\,\diamondsuit $), адаптировано из работы [55].
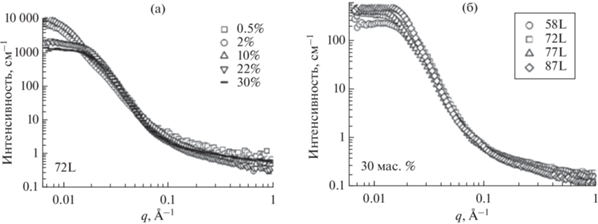
Характерный вид кривых не зависит от длины гидрофобного блока: кривые перекрываются во всем диапазоне векторов рассеяния, что свидетельствует об отсутствии взаимосвязи структуры и длины блока (рис. 5б) [55].
Крупномасштабные структуры с различной морфологией были обнаружены методами ультрамалоуглового рассеяния нейтронов и МУРН и подтверждены конфокальной микроскопией в работе Yin и соавт. (рис. 6) [53].
Рис. 6.
Изображения, полученные методом конфокальной микроскопии, для гидрогелей с различным L/D соотношением в гидрофобном блоке сополимера ПЛА-ПЭГ-ПЛА: (a) 50/50, (б) 75/25, (в) 95/5, (г) 100/0, адаптировано из работы [53].
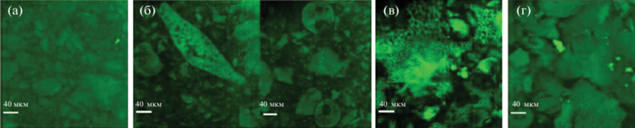
В другой работе Yin и соавт. гидрогели на основе блок-сополимеров П(D,L)ЛА-ПЭГ-П(D,L)ЛА с различным соотношением L/D были изучены методом широкоуглового рентгеновского рассеяния [43]. Было показано, что области ПЭГ в водном растворе стали аморфными, то есть характерные пики кристаллического ПЭГ (пик при q = 1.36 Å–1 и перекрывающиеся пики при q = 1.65–1.66 Å–1) не наблюдаются ни в одном из гелей. Кроме того, ни один из гелей не демонстрирует признаков стереокомплексообразования. Для образцов с соотношением 95/5 и 90/10 наблюдается пик от кристаллитов П(L)ЛА, которого нет на других кривых. С увеличением вклада L-изомера возрастает степень кристалличности системы.
В работе Mao и соавт. были исследованы стереокомплексные гидрогели, полученные путем смешения водных систем стереоизомерных двойных и тройных блок-сополимеров [56]. На кривых рентгеновского рассеяния в широких углах наблюдаются рефлексы от кристаллитов ПЛА при 11.9°, 13.5°, 15.4°, 18.0° (обозначены hc), пики ПЭГ отсутствуют, что указывает на гомокристаллическую природу ПЛА-доменов. Пики при 9.7°, 16.7°, 19.3° (обозначены sc) соответствуют стереокомплексным кристаллитам (рис. 7а).
Рис. 7.
(а) Широкоугловое рентгеновское рассеяние для стереокомплексных гидрогелей на основе сополимера ПЛА-ПЭГ-ПЛА; (б) малоугловое рентгеновское рассеяние для стереокомплексных гидрогелей на основе сополимера ПЛА-ПЭГ-ПЛА, адаптировано из работы [56].
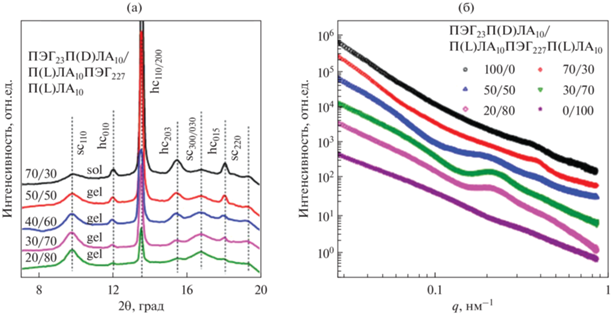
Двойные блок-сополимеры ПЛА-ПЭГ образуют “звездоподобные” мицеллы, тройные ПЛА-ПЭГ-ПЛА – “цветкоподобные”, смеси таких систем с различными конфигурациями гидрофобного блока дают мицеллы со стереомплексными ядрами (рис. 8а), а блок ПЛА может входить как в одну и ту же мицеллу, так и в две соседние. Однако на гелеобразование будет оказывать влияние не только формирование стереокомплексов, но и длина гидрофильного блока, а также массовое соотношение двойных и тройных блок-сополимеров. В случае короткого гидрофильного блока, размером меньше расстояния между ядрами мицелл, гелеобразования не происходит, т.к. оба блока ПЛА входят в одну мицеллу. При увеличении длины ПЭГ блоки ПЛА могу входить уже в соседние мицеллы с формированием мостиковой структуры, т.е. ПЛА-ПЭГ-ПЛА являются соединительными цепями между “звездоподобными” мицеллами ПЛА-ПЭГ, вследствие чего образуется физическая сетка гидрогеля. При дальнейшем увеличении длины блока ПЭГ наблюдается образование рыхлых слабо сшитых гидрогелей вследствие большого содержания гидрофильной компоненты. Массовый состав энантиомерной смеси также влияет на образование мостиковой структуры. При содержании ПЛА-ПЭГ-ПЛА менее 40–50 мас. % проходных цепей недостаточно для формирования гидрогеля (рис. 8б). Когда фракция тройного блок-сополимера превышает 80 мас. % в энантиомерной смеси преимущественно обнаруживаются “цветкоподобные” мицеллы. Из-за высокого содержания ПЭГ образование проходных цепей недостаточно для формирования гидрогеля. Наиболее благоприятным содержанием ПЛА-ПЭГ-ПЛА в смеси является 50–80 мас. % [56].
1.4. Механические свойства гидрогелей
Физико-механические свойства гидрогелей являются критически важной характеристикой, определяющей спектр их возможных применений. Основным недостатком гидрогелевых систем на основе блок-сополимеров ПЛА-ПЭГ является их невысокий уровень механических характеристик: модуль накоплений таких материалов, как правило, по результатам реологических исследований от температуры или частоты осцилляций не превышает 1 кПа [39, 42, 52, 54, 56, 57].
В работе [56] Mao с соавт. изучали механические свойства термочувствительных стереокомплексных гидрогелей – системы на основе смесей стереоизомерных двойных и тройных блок-сополимеров. Видно, что при низких температурах различные составы демонстрируют упруговязкие свойства, золь-гель переход к вязкоупругой жидкости происходит при температурах от 25 до 40°С (рис. 9а). На начальном участке модуль накоплений (G ') превалирует над модулем потерь (G ''), что говорит об образовании физически сшитого гидрогеля. Максимальный модуль накоплений порядка 1 кПа наблюдается для состава D/L 30/70 при температурах до 15°С (рис. 9б).
Рис. 9.
(а) Зависимость модулей накоплений (G '-закрашенные) и потерь (G ''-пустые) от температуры (частота 1 Гц) для стереокомплексной смеси П(D)ЛА-ПЭГ/П(L)ЛА-ПЭГ-П(L)ЛА; (б) фазовая диаграмма золь-гель перехода для стереокомплексного гидрогеля на основе стереокомплексной смеси П(D)ЛА-ПЭГ/П(L)ЛА-ПЭГ-П(L)ЛА (золь – пустые, гель – закрашенные), адаптировано из работы [56].
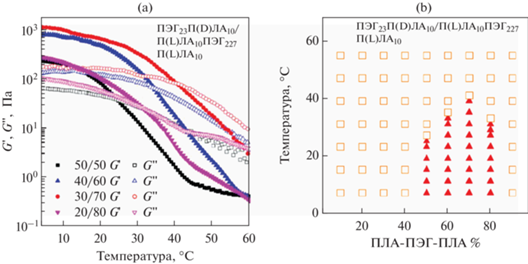
В работе [43] изучали реологию гидрогелей на основе П(D,L)ЛА-ПЭГ-П(D,L)ЛА с различным соотношением стереоизомеров L/D. Наблюдается слабая зависимость модуля накоплений (G ') от частоты осцилляций, значения G ' варьировались от 1 до 10 кПа в зависимости от соотношения, что является достаточно высоким для таких систем по данным литературы.
В другом исследовании изучены механические свойства гидрогелей на основе П(L)ЛА-ПЭГ-П(L)ЛА с различной длиной гидрофобного блока [58]. Были проведены испытания на одноосное сжатие, по полученным кривым напряжения от деформации установлены значения модуля упругости для исследуемых образцов. Следует отметить, что в большинстве научных трудов подобные материалы демонстрируют вязкое поведение и только в реологических испытаниях при высоких частотах наблюдается преобладание модуля накоплений (G ') над модулем потерь (G '') [39, 42, 52, 54, 56, 57]. В данной работе [58] материалы обладают упругостью без внешнего осцилляционного воздействия, могут быть исследованы на сжатие и дают рекордные значения модулей упругости для подобных систем (рис. 10). Такой результат можно объяснить длинами блоков сополимера и кристалличностью гидрофобного блока, вследствие чего происходит формирование более крупных и прочных агрегатов в физической сетке гидрогеля, что существенно повышает уровень механических характеристик.
Рис. 10.
Зависимость напряжения при сжатии от деформации для гидрогелей на основе сополимеров состава: L38P136L38 (1); L65P136L65 (2); L95P136L95 (3); L169P136L169 (4); L361P136L361 (5) [58].
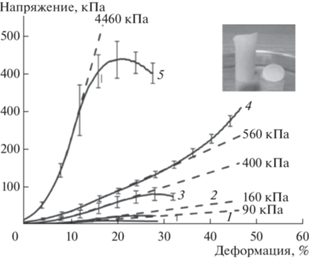
Физически сшитые гидрогели в большинстве научных трудов обладают слабыми механическими характеристиками, однако при наличии химических сшивок можно улучшить эти свойства. В работе Buwaldaс и соавт. звездообразные ПЭГ-П(D)ЛА и ПЭГ-П(L)ЛА модифицировали акрилатами и после сшивали под действием УФ-излучения [46]. Первоначально образованный физически сшитый гидрогель при концентрациях сополимера до 10 мас. % демонстрировал модуль накоплений не более 1–2 кПа, а от 10 до 20 мас. % модуль изменялся от 10 до 27 кПа. В случае химически сшитого материала даже при концентрациях менее 10 мас. % модуль накоплений был уже порядка 10 кПа, после 10 мас. % варьировался от 22 до 30 кПа.
1.5. Применение гидрогелей
Гидрогели на основе различных высокомолекулярных соединений – это коммерчески доступные материалы с широким спектром применений [1–10]. Однако помимо прочего такие изделия на основе биосовместимых и биоразлагаемых полимеров могут быть перспективными для использования в различных областях биомедицины в качестве средств доставки лекарственных препаратов, тканеинженерных конструкций и т.д. В настоящий момент активно ведутся исследования по расширению спектра их возможных применений. Одной из таких актуальных областей являются разработки по использованию материалов в терапии онкологических заболеваний [36, 38, 39, 52, 57, 59]. Применение цитотоксических противораковых препаратов имеет ряд недостатков, самый значительный из которых – неизбирательность, т.е. помимо опухолевых клеток, как правило, страдают здоровые органы и ткани. В связи с чем целевая доставка лекарства непосредственно в опухоль является решением данной проблемы.
В работе Darge с соавт. создали термочувствительный инъекционный гидрогель на основе П(D,L)ЛА-ПЭГ-П(D,L)ЛА и успешно импрегнировали в него два протироваковых препарата бевацизумаб и доксорубицин (DOX) [36]. Исследования in vitro показали низкую цитотоксичность самого гидрогеля. В экспериментах in vivo уже после однократного введения инъекции наблюдалось эффективное подавление опухоли без заметных повреждений жизненно важных органов.
В работе Liu с соавт. создали композитный термочувствительный гидрогель на основе ПЭГ-П(D,L)ЛА-ПЭГ для терапии меланомы [39]. В гидрогель загружали пористые ПЛА частицы с паклитакселом и цисплатин. Исследования in vitro показали, что полученный материал способен инициировать апоптоз опухолевых клеток и ингибировать их миграцию. In vivo на мышах-носителях меланомы был протестирован гидрогелевый композит. Установлено, что высвобождение лекарственных препаратов значительно ингибировало рост опухоли, не оказывая цитотоксического эффекта на здоровые органы и ткани.
В другой работе Yang с соавт. предложили комбинированную стратегию иммунотерапии рака [52]. Первоначально термочувствительный гидрогель на основе П(D,L)ЛА-ПЭГ-П(D,L)ЛА нагружали циклофосфамидом и вводили мышам, а через несколько дней делали еще одну инъекцию гидрогеля, содержащую цитозин-фосфат-гуаниновые нуклеотиды и лизаты опухолевых клеток для дальнейшего стимулирования противоопухолевых иммунных реакций. Такой подход успешно снижает токсический эффект от циклофосфамида на здоровые органы и ткани, при этом эффективно подавляет рост опухоли.
Среди других перспективных применений стоит отметить работу Zhao с соавт., где авторы предложили гидрогелевый глазной имплантат для терапии глаукомы – заболевания, характеризующегося повышенным внутриглазным давлением [54]. Был получен химически сшитый гидрогель на основе 4-лучевых ПЭГ с сильно разветвленной сетью, после чего его смешивали с мицеллами ПЭГ-ПЛА, в которые импрегнировали латанопрост и тимолол (рис. 11). Имплантат фиксируется в слезном протоке. Исследования in vitro показали увеличение действия препарата до 28 дней. Тесты на кроликах in vivo продемонстрировали эффект снижения внутриглазного давления в течение более чем 28 дней. Гистологические срезы и исследования по раздражающему действию имплантата на глаза показали достаточную безопасность материала.
Рис. 11.
Стадии разработки глазного имплантата на основе 4-лучевых ПЭГ, смешанного с мицеллами ПЭГ-ПЛА, адаптировано из работы [54].
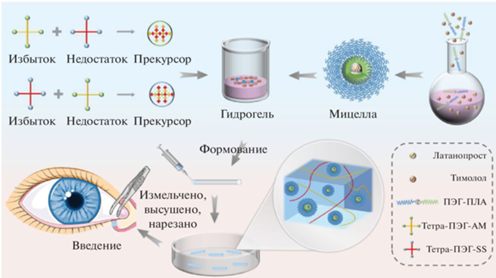
Yang с соавт. предложили использовать гидрогелевый материал на основе двойного блок-сополимера в качестве временного эмболизирующго средства [37]. Термочувствительный инъекционный гидрогель нагружали рентгеноконтрастным веществом иодопамином для возможности проведения интраоперационной рентгеновской визуализации. Также авторы считают, что гидрогель может быть использован как предоперационное эмболизирующее средство для удаления опухоли.
Pertici с соавт. применяли гидрогель на основе тройного блок-сополимера П(D,L)ЛА-ПЭГ-П(D,L)ЛА, модифицированного PNIPAAm, в качестве носителя лекарственных средств от нейродегенеративных патологий (болезнь Альцгеймера, болезнь Паркинсона, пароксизмальные дискинезии и т.д.) [47]. В гидрогель импрегнировали рилузол – нейропротекторное средство для лечения ишемии головного мозга и краситель цианин 5.5 для визуализации, и вводили в крыс. Была показана возможность инъекционного введения такого материала.
Grosjean с соавт. рассматривали в качестве тканевых клеев для лечения ран гидрогели на основе звездообразных ПЭГ-П(D,L)ЛА, функционализированные акрилатами, метакрилатами и пирокатехином [51]. Такой подход к закрытию ран имеет ряд преимуществ по сравнению с классическим наложением швов, т.к. сводится к минимуму повреждения тканей. В настоящее время наиболее часто применяются фибриновые и цианакрилатные биоадгезивы, однако они обладают некоторыми существенными недостатками: несмотря на высокие показатели адгезии, присутствует риск аллергических реакций, материалы могут быть токсичны, не разлагаемы. На модельных тканях было установлено, что производные акрилата и метакрилата достигают адгезионной прочности, близкой к таковой у цианакрилатных биоадгезивов (332 кПа), в свою очередь производные пирокатехина превосходят фибриновый клей (11 и 19 кПа). Низкий уровень цитотоксичности данных материалов подтверждает возможность их применения в качестве тканевых клеев.
Таким образом, гидрогели на основе сополимеров лактида и этиленгликоля обладают широким спектром перспективных применений благодаря своей биосовместимости и биоразлагаемости.
Согласно проведенному анализу, гидрогели на основе сополимеров лактида и этиленгликоля обладают наиболее высокими модулями упругости и при повышении температуры, как правило, претерпевают “гель–золь” переход.
2. ГИДРОГЕЛИ НА ОСНОВЕ СОПОЛИМЕРОВ ПОЛИЭТИЛЕНГЛИКОЛЯ И ПОЛИ(ЛАКТИД-СО-ГЛИКОЛИДА)
В отличие от рассмотренных выше сополимеров лактида и этиленгликоля, существенно большее количество работ посвящено исследованию температурно-индуцированного гелеобразования в растворах сополимеров на основе ПЭГ и поли(лактида-со-гликолида) (ПЛГА). При этом, как будет показано далее, особенности синтеза таких сополимеров определяют изменение механизма гелеобразования и фазового поведения относительно близких по химической структуре ПЭГ-ПЛА.
Материалы на основе сополимеров ПЭГ и ПЛ-ГА являются широко используемыми в различных областях медицины [60–62]. Данные соединения являются примером биоразлагаемых термочувствительных материалов, которые в водной среде за счет амфифильной природы способны образовывать мицеллярные агрегаты, а также гидрогели при изменении температуры. Благодаря различной природe молекул, входящих в состав ядра и оболочки, мицеллы и гидрогели могут включать как гидрофобные, так и гидрофильные лекарственные средства и обеспечивать их пролонгированное высвобождение.
2.1. Синтез блок-сополимеров
В целом, синтез сополимеров ПЭГ-ПЛГА неидентичен синтезу ПЭГ-ПЛА. Однако ввиду наличия гликолида, отличающегося по своей реакционной способности от лактида, достижение высоких молекулярных масс полиэфирных блоков затруднено. Вследствие этого усилия исследователей сосредоточены на реакциях с применением Sn(Oct)2 в качестве катализатора. Также не известны работы по получению разветвленных и звездообразных полиэфирных блоков.
Широко используемым способом получения блок-сополимеров является полимеризация с раскрытием цикла с использованием катализатора Sn(Oct)2 и макроинициатором ПЭГ (или метил-ПЭГ (мПЭГ)) (рис. 12) [20–22, 60–64].
Синтез сополимеров проводится как в растворе, так и в расплаве. На первом этапе ПЭГ осушают в расплаве под вакуумом. Далее перемещают ПЭГ в реакционный сосуд и добавляют заданные количества L- (или D-лактида (L-ЛА или D-ЛА) гликолидa (ГА) и Sn(Oct)2 и проводят реакцию полимеризации при перемешивании при 120–160°C в течение определенного времени. Варьируя количества ПЭГ, ГА и L-ЛА (или D-ЛА), регулируют длину цепи и соотношение блоков [20–22, 60–66]. Очищают полученный сополимер переосаждением в этилацетат или н-гексан, затем сушат под вакуумом при комнатной температуре [65]. Структурный анализ сополимеров имеет решающее значение как с теоретической, так и с промышленной точки зрения. При изучении сополимеров используют метод ядерно-магнитного резонанса (ЯМР), который предоставляет информацию о структуре и последовательности звеньев.
В работе [67] структурный анализ и оценку молекулярной массы сополимеров ПЛГА-ПЭГ-ПЛГА проводили с применением 1H-ЯМР-спектроскопии. Сигналы для ПЛГА-ПЭГ-ПЛГА находятся для CH (ЛА) на 5.1 ppm, CH3 (ЛА) на 1.45 ppm, CH2 (ГА) на 4.7 ppm, на 3.52 и 4.2 ppm – CH2 (этиленоксида). Аналогичным образом авторы [68] устанавливают структуру сополимеров, определяя соотношение ЛА/ГА звеньв и среднечисловую молекулярную массу (Mn) сополимеров ПЛ-ГА-ПЭГ-ПЛГА. Сигналы основных групп сополимера соотносятся с данными из работы [67]. Соотношения мономеров, которые использовали в процессе полимеризации, соответствовали соотношению ЛА к ГА в сополимере, рассчитанные с помощью 1H-ЯМР [68].
Тройные блок-сополимеры мПЭГ-ПЛГА-мПЭГ [69], привитые сополимеры ПЭГ-g-ПЛГА/ ПЛ-ГА-g-ПЭГ [70, 71] и звездообразные сополимеры ПЭГ с ПЛГА [72] получают полимеризацией соответствующих мономеров с раскрытием цикла вместе с реакциями прививки.
2.2. Получение гидрогелей
Сополимеры ПЛГА-ПЭГ способны набухать в водной среде с образованием золя при низких температурах и переходить в гель при нагревании [21]. Для того чтобы сополимеры с разнoй структурoй на основе ПЭГ-ПЛГА проявляли температурно-индуцированный фазовый переход в воде, необходимо соблюдать определенное соотношение между гидрофильным и гидрофобным блоками [73]. В работах [21, 64] подробно изучен процесс гелеобразования систем на основе сополимеров ПЭГ-ПЛГА. Были синтезированы тройные блок-сополимеры ПЛГА-ПЭГ-ПЛГА и двойные блок-сополимеры мПЭГ-ПЛГА c различными молекулярными массами (табл. 1) [21]. Растворы амфифильных блок-сополимеров были получены путем их растворения в воде при низких температурах, которые при нагревании оказались способны переходить в гель, дальнейшее повышение температуры приводило к образованию золя, а затем к коллапсу сополимеров и образованию осадка.
Таблица 1.
Молекулярные массы, синтезированных сополимеров, адаптировано из работы [21]
Блок-сополимер | Mn ПЭГ | Mn сополимера | Соотношение ЛА/ГА (моль/моль) |
Соотношение ПЛГА/ПЭГ (мас. %/мас. %) |
---|---|---|---|---|
ПЛГА-ПЭГ-ПЛГА | 1000 | 3420 | 2.4/1 | 2.4/1 |
ПЛГА-ПЭГ-ПЛГА | 1500 | 5240 | 2.5/1 | 2.5/1 |
ПЛГА-ПЭГ-ПЛГА | 2000 | 6980 | 2.4/1 | 2.5/1 |
мПЭГ-ПЛГА | 750 | 2630 | 2.5/1 | 2.5/1 |
Авторами был предложен механизм гелеобразования: самособирающаяся структура термогелеобразуемого сополимера ПЛГА-ПЭГ-ПЛГА в воде при низких температурах представляет собой мицеллы сферической формы. В водном растворе гидрофобные блоки ПЛГА в сополимере образуют ядро мицеллы за счет гидрофобных взаимодействий, а гидратированные блоки ПЭГ − гидрофильную оболочку. При этом возможно образование мостиковых структур между мицеллами, приводящее к образованию микрогелей. При повышении температуры термочувствительный ПЭГ теряет растворимость, и нарушается гидрофильная оболочка. Из-за изменения гидрофильно-гидрофобного баланса возникает нестабильное состояние, и мицеллы агрегируют, в результате чего образуется структура в виде связанных гидрофобных каналов ПЛА (рис. 13) [74]. Гидрогели на основе сополимеров ПЛГА-ПЭГ-ПЛГА обладают двумя состояниями гидрогелей, называемые гель-1 и гель-2. В первом случае возникают гидрофильныe мостики, то есть образуются проходныe цепи из ПЭГ между мицеллами, во втором случае формируются гидрофобные каналы за счет слияния ядер мицелл [22, 63]. В то же время отмечается, что гидрогели на основе двойных блок-сополимеров ПЭГ-ПЛГА и обратных тройных блок-сополимеров ПЭГ-ПЛГА-ПЭГ имеют только одно состояние геля, где точками сшивания является гидрофобные каналы [21].
Рис. 13.
Точки сшивания гидрогелей ПЛГА-ПЭГ-ПЛГА для состояний гель-1 и гель-2, адаптировано из работы [21].
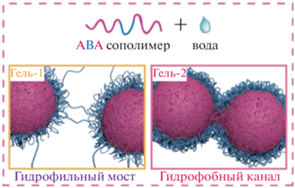
Такой золь–гель–золь переход характерен для всех сополимеров строения ПЛГА-ПЭГ-ПЛ-ГА [21, 22, 63–65, 75–79]. На фазовых диаграммах (рис. 14), полученных методом переворачивания виалов, представлены границы фазовых переходов золь–гель, где видно, что при увеличении концентрации сополимера в воде температура перехода золь–гель уменьшается, а температура перехода гель–золь, напротив, увеличивается.
Рис. 14.
Типичная фазовая диаграмма перехода золь-гель водного раствора тройных блок-сополимеров на основе ПЛГА-ПЭГ-ПЛГА, адаптировано из работы [65].
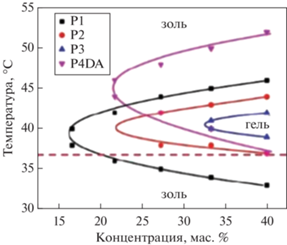
На золь–гель переход также оказывает влияние соотношение ЛА : ГА [78] в составе сополимера: снижение температуры фазового перехода из золя в гель и повышение температуры выпадения осадка происходит при увеличении молярного соотношения ЛА : ГА с 3 : 1 до 7 : 1 (рис. 15а). Такое поведение связано с увеличением концентрации мицелл. При этом рост содержания ПЭГ с 20 до 40% увеличивает температуру золь–гель перехода и снижает температуру выпадения осадка (рис. 15б) [78].
Рис. 15.
Фазовые диаграммы ПЛГА-ПЭГ-ПЛГА. (а) Влияние мольного соотношения лактид/ГА; (б) Влияние содержания ПЭГ [78].
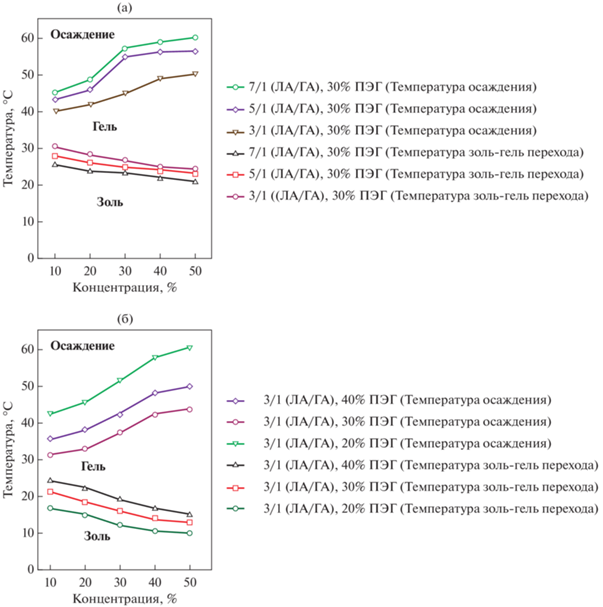
Гелеобразование систем на основе ПЭГ-ПЛГА изучают методaми реологии, исследуя зависимость модуля накоплений (G ') и модуля потерь (G '') от температуры [22, 62, 64, 65, 77, 80–82]. В работе [81] авторы изучили влияние лекарственного средства – имихимода (R837) и/или карбоната и хлорида кальция на температурно-индуцированное гелеобразование. Показано, что инкапсуляция лекарственного средства не помешала процессу гелеобразования, с повышением температуры значения модуля накоплений G ' начинали превалировать над значениями модуля потерь G ", что указывает на физическое гелеобразование при нагревании [81]. Авторы [65] комплексно охарактеризовали реологические свойства гидрогелей ПЛГА-ПЭГ-ПЛГА с модифицированными акриловой кислотой концевыми группами для УФ-сшивки. Гидрогели получали путем смешения тройных блок-сополимеров ПЛГА-ПЭГ-ПЛГА с модифицированными акрилоильными группами аналогичными сополимерами. На первом этапе проводили температурные испытания образцов гидрогелей, полученных на основе не модифицированных сополимеров, а далее полученные зависимости сравнивали с сополимерами с концевыми акрилоильными группами (рис. 16). В обоих случаях значения модулей накоплений и потерь заметно увеличиваются после 30°С, при этом наблюдается точка пересечения графиков изменения модулей G ' и G '', что свидетельствует о гелеобразовании. Дальнейшее увеличение температуры приводит к снижению значений модулей и появлению второй точки пересечения. Таким образом, при увеличении температуры в системе наблюдаются два перехода: золь–гель и гель–золь. Такая зависимость модулей от температуры является типичной для водных растворов сополимеров ПЛГА-ПЭГ-ПЛГА, способных к температурно-индуцированному гелеобразованию [21, 62, 65, 66, 76, 79, 81].
Рис. 16.
График зависимости модуля накоплений (G ') и модуля потерь (G '') от температуры для водных растворов на основе ПЛГА-ПЭГ-ПЛГА (частота 1 Гц), адаптировано из работы [65].
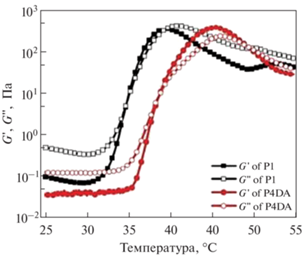
2.3. Структура и механические свойства гидрогелей
Практически для любого медицинского применения материалов на основе ПЛГА-ПЭГ важными характеристиками являются данные об их структуре и механических свойствах. В работе [65] были получены результаты реологических испытаний гидрогелей в зависимости от изменения угловой частоты. Частотные тесты и тиксотропные испытания проводили на гидрогелях, полученных смешением сополимеров с модифицированными и не модифицированными концевыми группами. Результаты показали, что механические и другие свойства гидрогелей можно легко регулировать, варьируя долю модифицированного полимера без ущерба для их переработки.
Были получены кривые течения – все образцы демонстрируют линейное снижение вязкости с увеличением скорости сдвига, что указывает на их истончение при сдвиге. А также изучена возможность восстановления структуры после прикладывания напряжения: при низкой деформации 1% G ' был выше, чем G '', что свидетельствует об упругом состоянии гидрогелей, тогда как резкое падение модуля наблюдалось в случае высокой деформации 100%. После снятия приложенного напряжения G ' гидрогелей мгновенно восстанавливается до исходных значений. Степень восстановления гидрогелей не изменяется как минимум после пяти циклов (рис. 17). Данный тест проводили так: на первом и третьем этапах в течение 100 с скорость сдвига была равной 0.1 с–1, на втором этапе в течение 5 с скорость сдвига составляла 100 с–1 [65].
Рис. 17.
Тиксотропный тест гидрогелей на основе ПЛГА-ПЭГ-ПЛГА. (а) Модуль накоплений (G ' − черный) и модуль потерь (G ''− красный) гидрогеля как функция времени. (б) Частотный тест. Модуль накоплений как функция угловой частоты, адаптировано из работы [65].
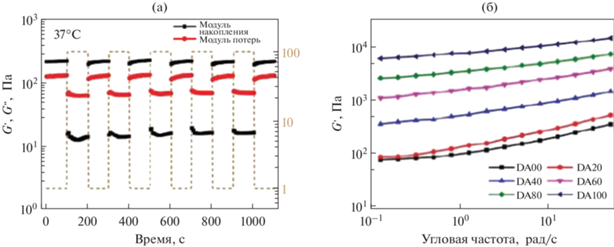
Реологический анализ был использован для паст на основе трикальцийфосфата (ТКФ) с добавкой термочувствительного биоразлагаемого сополимера ПЛГА-ПЭГ-ПЛГА [82]. Используемые в работе пасты застывают в течение 40 с после смешения жидкой и порошковой фаз. Добавление сополимера позволяет увеличить модуль накоплений и когезию материалов в первые секунды для возможности инъецировать материалы. Были изучены три системы: сополимер, паста α-ТКФ, смешанная с водой, и паста α-ТКФ с сополимером. Раствор сополимера демонстрирует асимптотическое увеличение напряжения сдвига и экспоненциальное снижение вязкости со скоростью сдвига. После снижения скорости сдвига наблюдается быстрое восстановление вязкого состояния (небольшая петля гистерезиса), что свидетельствует о псевдопластическом, а не тиксотропном реологическом поведении для сополимера. Для пасты αgТКФ, смешанной с водой и сополимером, петля гистерезиса имеет самый большой размер при разных порядках величины как напряжения сдвига, так и вязкости, что говорит о высокой степени тиксотропии (рис. 18). Материал с α-ТКФ, смешанный с сополимером, демонстрировал уменьшение напряжения сдвига с увеличением скорости сдвига после достижения максимальной точки, как и паста α-ТКФ/вода, но при этом сохранял большее значение при максимальной скорости сдвига и частичным восстановлением вязкости. Как было показано, использование сополимера в качестве добавки представляет интерес, поскольку обеспечивает улучшенные псевдопластичные свойства пасты на основе α-ТКФ и увеличивает ee вязкость за счет гелеобразования. Пасты поддерживают высокое напряжение сдвига при увеличении скорости сдвига, таким образом предотвращая разделение фаз во время введения [82].
Рис. 18.
Зависимость между напряжением сдвига и скоростью сдвига (а) и зависимость между вязкостью и скоростью сдвига (б) раствора сополимера 20 мас./об. % (синяя линия с треугольниками), пасты α-ТКФ с деионизированной водой (красная линия с кружками ) и пасты α-TКФ с раствором сополимера 20 мас./об. % (черная линия с квадратами), при 25°C. Стрелки указывают направление изменения скорости сдвига; петля гистерезиса указывает на тиксотропию, адаптировано из работы [82].
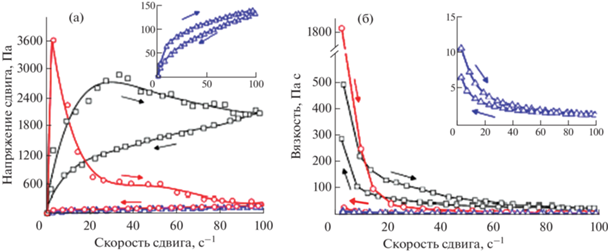
Морфологию системы на основе тройных блок-сополимеров оценивали на примере растворов с концентрацией 1% при помощи просвечивающего электронного микроскопа (ПЭМ) при различных температурах [21]. Подобранная концентрация ниже критической концентрации гелеобразования, но наблюдаемые типы морфологии все же могут в некоторой степени отражать эволюцию структуры материалов во время золь–гель перехода в концентрированной системе. Было показано, что молекулы сополимера образуют мицеллы при 25°C; формирование крупныx агрегатов с сетчатой структурой наблюдали при 37°С; при этом при 60°С образуются плотные агрегаты, макроскопически соответствующие осадку. В работе [83] также с использованием ПЭМ исследовали структуру ПЛГА-ПЭГ-ПЛГА, гидроксиапатита (ГАП) и композитного геля на основе ПЛГА-ПЭГ-ПЛГА/ГАП. Были получены данные, совпадающие с результатами других исследователей: в растворе ПЛГА-ПЭГ-ПЛГА образует мицеллы сферической формы (рис. 19) [21, 84].
Рис. 19.
ПЭМ-изображения водной системы сополимера ПЛГА-ПЭГ-ПЛГА при различных температурах, адаптировано из работы [21].
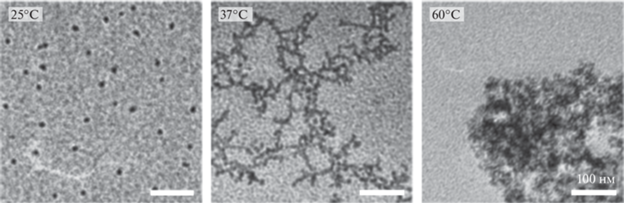
Для характеристики мицеллярной структуры тройных блок-сополимеров ПЛГА-ПЭГ-ПЛГА также применяют метод МУРН [85]. Полученная кривая МУРН была аппроксимирована с использованием модели сферической частицы со строением ядро-оболочка. Было подтверждено, что размер гидрофобного мицеллярного ядра зависит от молекулярной массы ПЛГА: c уменьшением молекулярной массы радиус гидрофобного ядра и мицеллы в целом уменьшаются. В работе [86] был обнаружен корреляционный пик в полуразбавленной системе сополимер–вода, что свидетельствует об упорядоченных структурах с характерным межмицеллярным расстоянием. При этом в области гелеобразования на фазовой диаграмме корреляционный пик исчезает − фор-мируется менее организованная структура. С увеличением температуры образуется цилиндрическая структура, а при еще более высоких температурах происходит беспорядочная упаковка цилиндров. В разбавленном растворе профили МУРН сополимерa с коротким ПЭГ-блоком имеют асимметричную (эллипсоидную) форму. Помимо структуры научная группа [86] оценивала показатель мутности гидрогелей. Для чувствительных к температуре блок-сополимеров определение мутности является ценным инструментом для исследования термодинамических изменений и агрегации в системе в зависимости от температуры. Повышение мутности может указывать на рост гидрофобных ассоциаций между мицеллами или самосборку цепей: более высокая концентрация способствует более частому столкновению мицелл, что приводит к образованию более крупныx ассоциативныx комплексов и повышенной мутности. Было показано, что при высоких температурах происходит резкое снижение мутности сополимеров, a амплитуда переходного пика более выражена для сополимеров с более коротким ПЭГ. Максимум мутности на фазовой диаграмме находится в области гидрогеля. При более высоких температурах мутность заметно снижается, что связано с разрушением гелевой сетки вследствие уплотнения гидрофобных микродоменов и структурной перестройки от связанных мицелл геля до уплотненных цилиндров [86].
2.4. Биоразложение гидрогелей
Важной характеристикой получаемых гидрогелей является их способность к биоразложению, так как они имеют широкий спектр применения в медицине [87]. В работе [88] были получены in situ термочувствительные гидрогели на основе тройного блок-сополимера ПЛГА-ПЭГ-ПЛГА, которые могут доставлять корилагин и низкомолекулярный хитозан (LC) непосредственно в опухолевую ткань. После инъекции раствор корилагина/LC/сополимера эффективно трансформируется в гидрогель в подкожных тканях мышей. Кроме этого, он полностью разрушается на 12-й день имплантации, что подтверждают исследования in vivo. Было показано, что после завершения терапии имплантированный гидрогель удаляется без хирургического вмешательства [88].
Авторы [89] исследовали как биосовместимость, так и биоразложение инъекционных имплантатов на основе ПЛГА-ПЭГ-ПЛГА с пролонгированной доставкой лекарственных средств. Нагруженный флуоресцином натрия термочувствительный гидрогель вводили в стекловидное тело глаза кролика, а затем регистрировали серию флуоресцентных изображений в определенные моменты времени для неинвазивного мониторинга внутриглазной ретенции сформированного in situ материала. Было показано, что матрица гидрогеля полностью разложилась за неделю, а прилегающие ткани не изменили обычную морфологию. Кроме того, ни в одном из исследований не наблюдалось признаков воспаления, некроза тканей или потери клеток эндотелия роговицы, что говорит о биосовместимости гидрогелевых материалов.
Для оценки биоразложения ученые [83] проводили как in vitro, так и in vivo исследования гелей на основе ПЛГА-ПЭГ-ПЛГА с ГАП. Материалы показали постепенную деградацию, на пятую неделю степень разложения составила свыше 90%. Эксперименты показали, что композитные гели с ГАП обладают свойствами биоразложения и биосовместимости. Однако стоит заметить, что для данных материалов возникала незначительная воспалительная реакция в подкожной клетчатке через 7 дней после имплантации [83].
2.5. Применение гидрогелей
Тройные блок-сополимеры ПЛГА-ПЭГ-П-ЛГА привлекают большое внимание благодаря простоте синтеза, воспроизводимости структуры, биобезопасности, термочувствительности и биоразлагаемости. Сополимеры и материалы на их основе подходят для многих областей применения: доставки противораковых, противобактериальных лекарственных средств, регенерации тканей и органов, лечения неоваскуляризации роговицы глаза, а также доставки инсулина для больных диабетом. За последние несколько лет спрос на термочувствительные сополимеры существенно вырос [90], а тройные блок-сополимеры ПЛГА-ПЭГ-ПЛГА являются основой для гидро-гелей различного применения. Так, мицеллы ПЛ-ГА-ПЭГ-ПЛГА с загруженным в них противораковым компонентом гексаметилендиамином 3β-ацетокси-урс-12-ен-28-овой кислоты (US597) были получены в работе [91] для лечения рака с длительной терапией. Было зафиксировано, что пиковая концентрация мицелл-US597 в плазме крови была почти в четыре раза выше по сравнению со свободным US597. Таким образом, мицеллы-US597 всасывались быстрее, чем свободный US597, у крыс, и степень всасывания, измеряемая по пиковой концентрации лекар-ственного средства в плазме, была значительно выше. Помимо этого, поменялась и фармакокинетика действующего вещества. Мицеллы-US597 имели в два раза более короткое время полувыведения, чем свободный US597 (8.716 ± 7.003 ч для мицелл-US597 и 16.433 ± 8.821 ч для US597), что указывает на то, что US597 выводился из плазмы крыс быстрее в случае мицелл-US597 по сравнению со свободным US597. Также было показано, что мицеллы-US597 характеризуются лучшей биодоступностью, чем свободное лекарственное средство. В совокупности эти данные свидетельствуют о том, что мицеллы-US597 обладают лучшей абсорбцией, метаболизмом и выведением, чем свободный US597, а также более длительным временем действия лекарственного средства, тем самым обладая более высокой растворимостью и противоопухолевой эффективностью. Увеличение концентрации и получение гидрогеля из подобной системы могут быть полезны при точечной доставке противоракового препарата.
Ученые [92] исследовали термочувствительный гель на основе ПЛГА-ПЭГ с включенным DOX. Была исследована противоопухолевая эффективность препаратов, содержащих DOX, на опухоли молочной железы у самок-мышей. Группы со свободным DOX, липосомами DOX, гелем DOX и липосомальным гелем, обогащенным DOX, имели показатели ингибирования опухоли 56.2, 59.7, 75.9 и 86.5% соответственно. Объем опухоли был значительно меньше в группах, получавших DOX не в свободном виде, следовательно, перитуморальная инъекция обладала более высокой противоопухолевой эффективностью. Липосомальный гель, содержащий DOX, имел самую высокую эффективность, что свидетельствует о наибольшей способности подавления опухоли, поскольку захваченное лекарственное средство должно проходить через липосомальный бислой и затем диффундировать из геля. Эти результаты предполагают, что гибридная система доставки лекарств, состоящая из липосом и гидрогелей ПЛГА-ПЭГ, может поддерживать высвобождение лекарств и улучшать их профиль высвобождения, а также повышать противоопухолевую эффективность за счет локализованной терапии и снижать цитотоксичность.
Доставка терапевтических лекарственных средств в задний сегмент глаза является сложной задачей из-за анатомии и физиологии глазных барьеров, поэтому становится актуальным подобрать способы доставки с пролонгированным действием. В работе [89] исследовали в качестве инъекционной матрицы гидрогели на основе ПЛГА-ПЭГ-ПЛГА с различным соотношением блоков. Мицеллы, образованные смесью сополимеров, способствовали солюбилизации гидрофобных молекул дексаметазона (DEX) в водной среде. С помощью парацентеза оценивали фармакокинетику после введения термочувствительного гидрогеля с DEX. В работе приведены профили высвобождения DEX, из которых видно, что препарат оставался обнаруживаемым в течение 9 дней после однократной инъекции гидрогеля. Также авторы отмечают, что начальная концентрация DEX, доставляемая в гелевой матрице, хорошо контролируется и составляет менее 10 мкг/мл в стекловидном теле. Эти данные свидетельствуют, что водный раствор сополимера быстро превращается в гидрогель в физиологической среде глаза после введения, и, таким образом, первая стадия высвобождения (взрывной эффект) нивелируется [89].
В работе [93] изучили гидрогели, полученные на основе смеси тройных блок-сополимеров поликапролактон-со-лактид-ПЭГ-поликапролактон-со-лактид (ПКЛА-ПЭГ-ПКЛА) и ПЛГА-ПЭГ-ПЛГА. Микросферы ПЛГА использовали для инкапсулирования эксенатида, которые затем заключали в гидрогели. Инъекция приготовленной выше композиции приводит к стабильной концентрации глюкозы в крови у обработанных мышей, а также к хорошему сохранению массы тела в течение 20 дней в фармакодинамическом исследовании. Эффекты микросфер и гидрогеля с микросферами и эксенатидом сравнивали с помощью перорального глюкозотолерантного теста на 20-й день после инъекции. Результаты показали, что в группе, получавшей гидрогель с частицами, сохранялось высокое биоактивное высвобождение эксенатида, тогда как в группе, которая получала только микросферы, оно прекращалось ранее. Таким образом, было установлено, что гидрогель с микросферами, нагруженный эксенатидом, является потенциально возможным препаратом длительного действия для лечения диабета.
В другом исследовании [94] синтезировали гидрогели на основе ПЛГА-ПЭГ-ПЛГА, в которые вводили симвастатин. Свойства гидрогелей были исследованы in vivo с использованием модели костного дефекта крысы. По сравнению с контрольными образцами, эксперименты с использованием гидрогелей ПЛГА-ПЭГ-ПЛГА с симвастатином демонстрировали высокую скорость роста новой костной ткани. Профили высво-бождения препарата из гелей in vitro показали замедленное высвобождение симавастина: концентрация высвободившегося препарата лишь на 14 сут достигала приблизительно 80%. Таким образом, результаты этого исследования показали, что симвастатин/ПЛГА-ПЭГ-ПЛГА может иметь терапевтические перспективы для восстановления костной ткани [94].
В работе [95] создали систему доставки инсулина путем включения наночастиц хитозана в гидрогель ПЛГА-ПЭГ-ПЛГА (ICNPH). Субконъюнктивальное введение гидрогеля уменьшало амплитуду скотопической B-волны, устраняло микро- и ультраструктурные нарушения в сетчатке и уменьшало апоптоз клеток сетчатки у крыс с диабетической ретинопатией. В результате экспериментов с различными группами животных, в том числе с контрольными группами, было доказано, что субконъюнктивальная инъекция IC-NPH оказывала значительное нейропротекторное воздействие на сетчатку у крыс с диабетической ретинопатией и способствовала регулируемой доставке инсулина [95].
Таким образом, сополимеры ПЭГ-ПЛГА, как правило, имеют невысокие молекулярные массы, и за счет этого способны к “золь–гель–золь” переходу.
ЗАКЛЮЧЕНИЕ
Гидрогелевые материалы на основе сополимеров биоразлагаемых полиэфиров и ПЭГ являются перспективными во многих областях биомедицины. Наблюдается неугасающий интерес к данным системам ввиду резорбируемости и биосовместимости полимерной сетки, а также из-за возможности точно “настраивать” механические свойства и структуру гидрогелей. За счет возможности синтеза сополимеров этиленгликоля с лактидом с относительно высокими молекулярными массами, гидрогели на их основе, как правило, имеют “гель–золь” переход и не обладают термочувствительностью. Такие материалы могут мыть использованы в качестве имплантатов мягких тканей. При этом, как показано в обзоре, присутствует ряд работ, где всe же используются термочувствительные гидрогели на основе сополимеров ПЭГ-ПЛА низкой молекулярной массы. Гораздо большее число исследований термочувствительных гидрогелей проведено для систем ПЭГ-ПЛГА. Гели на основе данных сополимеров имеют “золь–гель–золь” переход и могут быть использованы для доставки лекарств. В то же время существуют вопросы, требующие дополнительных исследований в данной области: подбор эффективных безопасных катализаторов для синтеза сополимеров, детальные исследования механизмов гелеобразования, получение материалов с высокими модулями упругости и др.
БЛАГОДАРНОСТИРабота выполнена при финансовой поддержке ФГБНУ РНЦХ им. акад. Б.В. Петровского (договор № 769-ЭА-23-НИР от 26.06.23).
Список литературы
Максимова Ю.Г., Щетко В.А, Максимов А.Ю. Полимерные гидрогели в сельском хозяйстве (обзор) // Сельскохозяйственная биология. 2023. Т. 58. № 1. С. 23–42. https://doi.org/10.15389/agrobiology.2023.1.23rus
Kaur P., Agrawal R., Pfeffer F.M. et al. Hydrogels in agriculture: Prospects and challenges // Journal of Polymers and the Environment. 2023. V. 35. P. 3701–3718. https://doi.org/10.1007/s10924-023-02859-1
Асулян Л.Д., Бояркина В.В, Агаева М.В. Гидрогели поливинилового спирта как влагоудерживающие сорбенты // Известия ТулГУ. Естественные науки. 2021. № 1. С. 13–19. https://doi.org/10.24412/2071-6176-2021-1-13-19
Рабаданов Р.Г., Рабаданов Г.Г., Мукаилов М.Д., Атаев М.З. Сильнонабухающие полимерные гидрогели на плодоносящих виноградниках Южного Дагестана // Проблемы развития АПК региона. 2017. Т. 29. № 1(29). С. 46–52.
Tran N-P-D., Yang M-C., Tran-Nguyen P.L. Evaluation of silicone hydrogel contact lenses based on poly(dimethylsiloxane) dialkanol and hydrophilic polymers // Colloids and Surfaces B: Biointerfaces. 2021. V. 206. P. 111957. https://doi.org/10.1016/j.colsurfb.2021.111957
Бондаренко П.И., Пинчук Л.С., Дравица Л.В., Бондаренко Н.Ю. Лечебные контактные линзы и способы их изготовления (обзор) // Офтальмология. Восточная Европа. 2011. № 3(10). С. 78–91.
Мельник С.И., Торикашвили В.Д., Якута К.Д., Лебедева С.А. Раневые повязки и мягкие лекарственные формы на основе коллагена для лечения ран различной этиологии // Фармацевтическое дело и технология лекарств. 2020. № 6. С. 10–16. https://doi.org/10.33920/med-13-2006-01
Кузнецова Т.А., Беседнова Н.Н., Усов В.В., Андрюков Б.Г. Биосовместимые и биодеградируемые раневые покрытия на основе полисахаридов из морских водорослей (обзор литературы) // Вестник хирургии им. И.И. Грекова. 2020. Т. 179. № 4. С. 109–115. https://doi.org/10.24884/0042-4625-2020-179-4-109-115
Дуданов И.П., Виноградов В.В., Криштоп В.В., Никонорова В.Г. Преимущества и недостатки гелевых покрытий в терапии ожоговых ран и ожогов (обзор литературы) // Вестник новых медицинских технологий. Электронное издание. 2022. Т. 16. № 2. С. 13–22. https://doi.org/10.24412/2075-4094-2022-2-1-2
Ушмаров Д.И., Гуменюк А.С., Гуменюк С.Е. и др. Сравнительная оценка многофункциональных раневых покрытий на основе хитозана: многоэтапное рандомизированное контролируемое экспериментальное исследование // Кубанский научный медицинский вестник. 2021. Т. 28. № 3. С. 78–96. https://doi.org/10.25207/1608-6228-2021-28-3-78-96
Glukhova S.A., Molchanov V.S., Kharitonova E.P. et al. Green nanocomposite gels based on binary network of sodium alginate and percolating halloysite clay nanotubes for 3D printing // Carbohydrate Polymers. 2022. V. 282. P. 119106. https://doi.org/10.1016/j.carbpol.2022.119106
Григорьев А.М., Басок Ю.Б., Кириллова А.Д. и др. Криогенно-структурированный гидрогель на основе желатина как резорбируемая макропористая матрица для биомедицинских технологий // Вестник трансплантологии и искусственных органов. 2022. Т. 24. № 2. С. 83–93. https://doi.org/10.15825/1995-1191-2022-2-83-93
Osidak E.O., Andreev A.Yu., Avetisov S.E. et al. Corneal stroma regeneration with collagen-based hydrogel as an artificial stroma equivalent: A comprehensive in vivo study // Polymers. 2022. V. 14. № 19. P. 4017. https://doi.org/10.3390/polym14194017
Васильев А.В., Кузнецова В.С., Галицына Е.В. и др. Биосовместимость и остеогенные свойства коллаген-фибронектинового гидрогеля, импрегнированного BMP-2 // Стоматология. 2019. Т. 98. № 6(2). С. 5–11. https://doi.org/10.17116/stomat2019980625
Шилова С.В., Миргалеев Г.М., Волкова М.В. и др. Биосовместимые системы доставки антибиотика цефотаксима на основе гелевых микрочастиц альгината кальция // Вестник Технологического университета. 2021. Т. 24. № 12. С. 56–59.
Yermak I.M., Gorbach V.I., Karnakov I.A., Davydova V.N. et al. Carrageenan gel beads for echinochrome inclusion: Influence of structural features of carrageenan // Carbohydrate Polymers. 2021. V. 272. P. 118479. https://doi.org/10.1016/j.carbpol.2021.118479
Vasilyev A.V., Kuznetsova V.S., Bukharova T.B. et al. Influence of the degree of deacetylation of chitosan and BMP-2 concentration on biocompatibility and osteogenic properties of BMP-2/PLA granule-loaded chitosan/β-glycerophosphate hydrogels // Molecules. 2021. V. 26. № 2. P. 261. https://doi.org/10.3390/molecules26020261
Wang Z., Ye Q., Yu S., Akhavan B. Poly ethylene glycol (PEG)-based hydrogels for drug delivery in cancer therapy: A comprehensive review // Advanced Healthcare Materials. 2023. V. 12. № 18. P. 2300105. https://doi.org/10.1002/adhm.202300105
Бакеева И.В., Докторова А.В., Дамшкалн Л.Г., Лозинский В.И. Криоструктурирование полимерных систем. 54. Гибридные органо-неорганические криогели поливинилового спирта, наполненные образующимся in situ // Коллоидный журнал. 2021. Т. 83. № 1. С. 35–50. https://doi.org/10.31857/S002329122101002X
Jeong B., Wang L.Q., Gutowska A. Biodegradable thermoreversible gelling PLGA-g-PEG copolymers† // Chemical Communications. 2001. № 16. P. 1516–1517. https://doi.org/10.1039/B102819G
Cui S., Yu L., Ding J. Thermogelling of amphiphilic block copolymers in water: ABA type versus AB or BAB type // Macromolecules. 2019. V. 52. P. 3697−3715. https://doi.org/10.1021/acs.macromol.9b00534
Yu L., Ding J. Injectable hydrogels as unique biomedical materials // Chemical Society Reviews. 2008. V. 37. P. 1473–1481. https://doi.org/10.1039/B713009K
Kricheldorf H.R., Meier-Haack J. Polylactones, 22† ABA triblock copolymers of L-lactide and poly(ethylene glycol) // Macromolecular Chemistry. 1993. V. 194. № 2. P. 715–725. https://doi.org/10.1002/macp.1993.021940229
Deng X.M., Xu R.P., Xiong C.D., Cheng L.M. Synthesis and characterization of block copolymers from D,L-lactide and poly(ethylene glycol) with stannous chloride // Journal of Polymer Science Part C: Polymer Letters. 1990. V. 28. № 13. P. 411–416. https://doi.org/10.1002/pol.1990.140281303
Kissel. T., Li Y.X., Volland C. Properties of block- and random-copolymers of lactic acid and glycolic acid // Proc. Int. Symp. Controlled Release Bioact. Mater. 1993. P. 127–128.
Youxin L., Kissel T. Synthesis and properties of biodegradable ABA triblock copolymers consisting of poly (L-lactic acid) or poly (L-lactic-co-glycolic acid) A-blocks attached to central poly (oxyethylene) B-blocks // Journal of Controlled Release. 1993. V. 27. № 3. P. 247–257. https://doi.org/10.1016/0168-3659(93)90155-X
Stevels W.M., Ankone M.L.K., Dijkstra P.J., Feijen J. Kinetics and mechanism of L-lactide polymerization using two different yttrium alkoxides as initiators // Macromolecules. 1996. V. 29. № 19. P. 6132–6138. https://doi.org/10.1021/ma9605311
Stevels W.M., Ankone M.L.K., Dijkstra P.J., Feijen J. A versatile and highly efficient catalyst system for the preparation of polyesters based on lanthanide tris(2,6-di-tert-butylphenolate)s and various alcohols // Macromolecules. 1996. V. 29. № 9. P. 3332–3333. https://doi.org/10.1021/ma951813o
Li S., Anjard S., Rashkov I., Vert M. Hydrolytic degradation of PLA/PEO/PLA triblock copolymers prepared in the presence of Zn metal or CaH2 // Polymer. 1998. V. 39. № 22. P. 5421–5430. https://doi.org/10.1016/S0032-3861(97)10272-5
Cerrai P., Tricoli M. Block copolymers from L-lactide and polyethylene glycol through a non-catalyzed route // Macromolecular Chemistry Rapid Communications. 1993. V. 9. № 9. P. 529–538. https://doi.org/10.1002/marc.1993.030140901
Sanabria-DeLong N., Agrawal S.K., Bhatia S.R., Tew G.N. Impact of synthetic technique on PLA-PEO-PLA physical hydrogel properties // Macromolecules. 2007. V. 40. № 22. P. 7864–7873. https://doi.org/10.1021/ma071243f
Hamia M., Aminib M. et al. Synthesis and in vitro evaluation of a pH-sensitive PLA–PEG–folate based polymeric micelle for controlled delivery of docetaxel // Colloids and Surfaces B: Biointerfaces. 2014. V. 116. P. 309–317. https://doi.org/10.1016/j.colsurfb.2014.01.015
Subbu S. Venkatraman., Pan Jie et al. Micelle-like nanoparticles of PLA–PEG–PLA triblock copolymer as chemotherapeutic carrier // Pharmaceutical Nanotechnology. 2005. V. 298. № 1. P. 219–232. https://doi.org/10.1016/j.ijpharm.2005.03.023
Li L., Cao Z.-Q. et al. Poly(L-lactic acid)-polyethylene glycol-poly(L-lactic acid) triblock copolymer: A novel macromolecular plasticizer to enhance the crystallization of poly(L-lactic acid) // Europian Polymer Journal. 2017. V. 97. P. 272–281. https://doi.org/10.1016/j.eurpolymj.2017.10.025
Qin W., Chuandong W. et al. Synthesis, thermosensitive gelation and degradation study of a biodegradable triblock copolymer // Journal of Macromolecular Science. 2013. V. 50. № 2. P. 200–207. https://doi.org/10.1080/10601325.2013.742794
Darge H.F., Andrgie A.T. et al. Localized controlled release of bevacizumab and doxorubicin by thermo-sensitive hydrogel for normalization of tumor vasculature and to enhance the efficacy of chemotherapy // International Journal of Pharmaceutics. 2019. V. 575. P. 118799. https://doi.org/10.1016/j.ijpharm.2019.118799
Yang H., Lei K. et al. Injectable PEG/polyester thermogel: A new liquid embolization agent for temporary vascular interventional therapy // Materials Science & Engineering C. 2019. V. 102. P. 606–615. https://doi.org/10.1016/j.msec.2019.04.075
Darge H.F., Andrgie A.T. et al. Multifunctional drug-loaded micelles encapsulated in thermo-sensitive hydrogel for in vivo local cancer treatment: Synergistic effects of anti-vascular and immuno-chemotherapy // Chemical Engineering Journal. 2021. V. 406. P. 126879. https://doi.org/10.1016/j.cej.2020.126879
Liu. Y., Ma W. et al. In situ administration of temperature-sensitive hydrogel composite loading paclitaxel microspheres and cisplatin for the treatment of melanoma // Biomedicine & Pharmacotherapy. 2023. V. 160. P. 114380. https://doi.org/10.1016/j.biopha.2023.114380
Tanzi M.C., Verderio P. et al. Cytotoxicity of some catalysts commonly used in the synthesis of copolymers for biomedical use // Journal of Materials Science: Materials in Medicine. 1994. V. 5. P. 393–396. https://doi.org/10.1007/BF00058971
Chen G.X., Kim H.S. et al. Synthesis of high-molecular-weight poly(L-lactic acid) through the direct condensation polymerization of L-lactic acid in bulk state // European Polymer Journal. 2006. V. 42. № 2. P. 468–472. https://doi.org/10.1016/j.eurpolymj.2005.07.022
Fenton O. S., Tibbitt M.W. et al. Injectable polymer−nanoparticle hydrogels for local immune cell recruitment // Biomacromolecules. 2019. V. 20. № 12. P. 4430–4436. https://doi.org/10.1021/acs.biomac.9b01129
Yin X., Hewitt D.R.O. et al. Impact of stereochemistry on rheology and nanostructure of PLA–PEO–PLA triblocks: Stiff gels at intermediate L/D-lactide ratios // Soft Matter. 2018. V. 14. № 35. P. 7255–7263. https://doi.org/10.1039/C8SM01559G
Yin X., Hewitt D.R.O. et al. Effect of stereochemistry on nanoscale assembly of ABA triblock copolymers with crystallizable blocks // Polymer. 2021. V. 223. P. 123683. https://doi.org/10.1016/j.polymer.2021.123683
Mhiri S., Abid M. et al. Green synthesis of covalent hybrid hydrogels containing PEG/PLA‑based thermoreversible networks // Journal of Polymer Research. 2022. V. 29. № 8. P. 328. https://doi.org/10.1007/s10965-022-03153-9
Buwalda S.J., Dijkstra P.J. et al. In situ forming stereocomplexed and post-photocrosslinked acrylated star poly(ethylene glycol)-poly(lactide) hydrogels // European Polymer Journal. 2017. V. 94. P. 152–161. https://doi.org/10.1016/j.eurpolymj.2017.07.002
Pertici V., Pinbarre C. et al. Degradable and injectable hydrogel for drug delivery in soft tissues // Biomacromolecules. 2018. V. 20. № 1. P. 149–163. https://doi.org/10.1021/acs.biomac.8b01242
Heskins M., Guillet J.E. Solution properties of poly(N-isopropylacrylamide) // Journal of Macromolecular Science: Part A. Chemistry. 1968. V. 2. № 8. P. 1441–1455. https://doi.org/10.1080/10601326808051910
Fujishige S., Kubota K., Ando I. Phase transition of aqueous solutions of poly(N-isopropylacrylamide) and poly(N-isopropylmethacrylamide) // Journal of Physical Chemistry. 1989. V. 93. № 8. P. 3311–3313. https://doi.org/10.1021/j100345a085
Trinh T.A., Le T.M.D. et al. A novel injectable pH–temperature sensitive hydrogel containing chitosan–insulin electrosprayed nanosphere composite for an insulin delivery system in type I diabetes treatment // Biomaterials. 2020. V. 8. № 14. P. 3830–3843. https://doi.org/10.1039/D0BM00634C
Grosjean M., Girard E. et al. Degradable bioadhesives based on star PEG−PLA hydrogels for soft tissue applications // Biomacromolecules. 2022. https://doi.org/10.1021/acs.biomac.2c01166
Yang F., Shi K., Hao Y. et al. Cyclophosphamide loaded thermo-responsive hydrogel system synergize with a hydrogel cancer vaccine to amplify cancer immunotherapy in a prime-boost manner // Bioactive Materials. 2021. V. 6. № 10. P. 3036–3048. https://doi.org/10.1016/j.bioactmat.2021.03.003
Yin X., Hewitt D.R. et al. Hierarchical assembly in PLA-PEO-PLA hydrogels with crystalline domains and effect of block stereochemistry // Colloids and Surfaces B: Biointerfaces. 2019. V. 180. P. 102–109. https://doi.org/10.1016/j.colsurfb.2019.04.031
Zhao J., Xiong J. et al. A triple crosslinked micelle-hydrogel lacrimal implant for localized and prolonged therapy of glaucoma // European Journal of Pharmaceutics and Biopharmaceutics. 2023. V. 185. P. 44–54. https://doi.org/10.1016/j.ejpb.2023.02.011
Agrawal S.K., Sanabria-DeLong N. et al. Structural characterization of PLA-PEO-PLA solutions and hydrogels: Crystalline vs amorphous PLA domains // Macromolecules. 2008. V. 41. № 5. P. 1774–1784. https://doi.org/10.1021/ma070634r
Mao H., Pan P. et al. In situ formation and gelation mechanism of thermoresponsive stereocomplexed hydrogels upon mixing diblock and triblock poly(lactic acid)/poly(ethylene glycol) copolymers // The Journal of Physical Chemistry. 2015. V. 119. № 21. P. 6471–6480. https://doi.org/10.1021/acs.jpcb.5b03610
Yang. F., Shi K. et al. A biodegradable thermosensitive hydrogel vaccine for cancer immunotherapy // Applied Materials Today. 2020. V. 19. P. 100608. https://doi.org/10.1016/j.apmt.2020.100608
Загоскин Ю.Д., Григорьев Т.Е. и др. Гидрогели и губчатые материалы на основе тройных блок-сополимеров лактида и этиленгликоля // Доклады академии наук. 2019. Т. 486. № 4. С. 433–436. https://doi.org/10.1134/S001250081906003X
Gholizadeh H., Landh E., Silva D.M. et al. In vitro and in vivo applications of a universal and synthetic thermo-responsive drug delivery hydrogel platform // International Journal of Pharmaceutics. 2023. V. 635. P. 122777. https://doi.org/10.1016/j.ijpharm.2023.122777
Chen S., Pieberb R. et al. Triblock copolymers: Synthesis, characterization, and delivery of a model protein // International Journal of Pharmaceutics. 2005. V. 288. № 2. P. 207–218. https://doi.org/10.1016/j.ijpharm.2004.09.026
Qiao M., Chen D. et al. Injectable biodegradable temperature-responsive PLGA–PEG–PLGA copolymers: Synthesis and effect of copolymer composition on the drug release from the copolymer-based hydrogels // International Journal of Pharmaceutics. 2005. V. 294. № 1–2. P. 103–112. https://doi.org/10.1016/j.ijpharm.2005.01.017
Yu L., Xu W. et al. Poly(lactic acid-co-glycolic acid)–poly(ethylene glycol)–poly(lactic acid-co-glycolic acid) thermogel as a novel submucosal cushion for endoscopic submucosal dissection // Acta Biomaterialia. 2014. V. 10. № 3. P. 1251–1258. https://doi.org/10.1016/j.actbio.2013.12.007
Chen L., Ci T., Li T. et al. Effects of molecular weight distribution of amphiphilic block copolymers on their solubility, micellization, and temperature-induced sol−gel transition in water // Macromolecules. 2014. V. 47. № 17. P. 5895–5903. https://doi.org/10.1021/ma501110p
Chen L., Ci T., Yu L. et al. Effects of molecular weight and its distribution of PEG block on micellization and thermogellability of PLGA−PEG−PLGA copolymer aqueous solutions // Macromolecules. 2015. V. 48. № 11. P. 3662–3671. https://doi.org/10.1021/acs.macromol.5b00168
Zhou Y., Cui Y., Wang L.Q. A Dual-sensitive hydrogel based on poly(lactide-co-glycolide)-polyethylene glycol-poly(lactide-co-glycolide) block copolymers for 3D printing // International Journal of Bioprinting. 2021. V. 7. № 3. P. 140–152. https://doi.org/10.18063/ijb.v7i3.389
Zentner G., Rathi R., Shih C. et al. Biodegradable block copolymers for delivery of proteins and water-insoluble drugs // Journal of Controlled Release. 2001. V. 72. № 1–3. P. 203–215. https://doi.org/10.1016/S0168-3659(01)00276-0
Ghahremankhani A., Dorkoosh F., Dinarvand R. PLGA-PEG-PLGA tri-block copolymers as in situ gel-forming peptide delivery system: Effect of formulation properties on peptide release // Pharmaceutical Development and Technology. 2008. V. 13. № 1. P. 49–55. https://doi.org/10.1080/10837450701702842
Khodaverdi E. Tekie F. et al. Preparation and investigation of sustained drug delivery systems using an injectable, thermosensitive, in situ forming hydrogel composed of PLGA–PEG–PLGA // AAPS PharmSciTech. 2012. V. 13. P. 590–600. https://doi.org/10.1208/s12249-012-9781-8
Jeong B., Bae Y.H., Kim S.W. Thermoreversible gelation of PEG-PLGA-PEG triblock copolymer aqueous solutions // Macromolecules. 1999. V. 32. № 21. P. 7064–7069. https://doi.org/10.1021/ma9908999
Jeong B., Lee K.M., Gutowska A., An Y.H. %Thermogelling biodegradable copolymer aqueous solutions for injectable protein delivery and tissue engineering // Biomacromolecules. 2002. V. 3. № 4. P. 865–868. https://doi.org/10.1021/bm025536m
Chung Y.-M., Simmons K.L., Gutowska A., Jeong B. Sol-gel transition temperature of PLGA-g-PEG aqueous solutions // Biomacromolecules. 2002. V. 3. № 3. P. 511–516. https://doi.org/10.1021/bm0156431
Lee S.J., Han B.R., Park S.Y. et al. Sol–gel transition behavior of biodegradable three-arm and four-arm star-shaped PLGA–PEG block copolymer aqueous solution // Journal of Polymer Science: Part A: Polymer Chemistry. 2006. V. 44. № 2. P. 888–899. https://doi.org/10.1002/pola.21193
Shi J., Yu L., Ding J. PEG-based thermosensitive and biodegradable hydrogels // Acta Biomaterialia. 2021. V. 128. P. 42–59. https://doi.org/10.1016/j.actbio.2021.04.009
Cui S., Yu L., Ding J. Semi-bald micelles and corresponding percolated micelle networks of thermogels // Macromolecules. 2018. V. 51. № 16. P. 6405–6420. https://doi.org/10.1021/acs.macromol.8b01014
Lopez-Cano J.J., Sigen A., Andrés-Guerrero V. et al. Thermo-responsive PLGA-PEG-PLGA hydrogels as novel injectable platforms for neuroprotective combined therapies in the treatment of retinal degenerative diseases // Pharmaceutics. 2021. V. 13. № 2. P. 234. https://doi.org/10.3390/pharmaceutics13020234
Wei P-S., Chen Y.J., Lin S.Y. et al. In situ subcutaneously injectable thermosensitive PEG-PLGA diblock and PLGA-PEG-PLGA triblock copolymer composite as sustained delivery of bispecific anti-CD3 scFv T‑cell/anti-EGFR Fab Engager (BiTEE) // Biomaterials. 2021. V. 278. P. P. 121166. https://doi.org/10.1016/j.biomaterials.2021.121166
Cespi M., Bonacucina G., Tiboni M. et al. Insights in the rheological properties of PLGA-PEG-PLGA aqueous dispersions: Structural properties and temperature-dependent behavior // Polymer. 2021. V. 213. P. 123216. https://doi.org/10.1016/j.polymer.2020.123216
Kamali H., Khodaverdi E., Hadizadeh F. et al. Comparison of in-situ forming composite using PLGA-PEG-PLGA with in-situ forming implant using PLGA: In-vitro, ex-vivo, and in-vivo evaluation of naltrexone release // Journal of Drug Delivery Science and Technology. 2019. V. 50. P. 188–200. https://doi.org/10.1016/j.jddst.2019.01.011
Chen X., Wang H. et al. An injectable and active hydrogel induces mutually enhanced mild magnetic hyperthermia and ferroptosis // Biomaterials. 2023. V. 298. P. 122139. https://doi.org/10.1016/j.biomaterials.2023.122139
Steinman N.Y, Haim-Zada M. et al. Effect of PLGA block molecular weight on gelling temperature of PL-GA-PEG-PLGA thermoresponsive copolymers // Journal of Polymer Science Part A: Polymer Chemistry. 2019. V. 57. № 1. P. 35–39. https://doi.org/10.1002/pola.29275
Cao D., Guo W., Cai C. et al. Unified therapeutic-prophylactic vaccine demonstrated with a postoperative filler gel to prevent tumor recurrence and metastasis // Advanced Functional Materials. 2022. V. 32. № 40. P. 2206084. https://doi.org/10.1002/adfm.202206084
Vojtova L., Michlovska L., Valova K. et al. The effect of the thermosensitive biodegradable PLGA–PEG–PLGA copolymer on the rheological, structural and mechanical properties of thixotropic self-hardening tricalcium phosphate cement // International Journal of Molecular Science. 2019. V. 20. № 2. P. 391. https://doi.org/10.3390/ijms20020391
Yuan B., Zhang Y., Wang Q. et al. Thermosensitive vancomycin@PLGA-PEG-PLGA/HA hydrogel as an all-in-one treatment for osteomyelitis // International Journal of Pharmaceutics. 2022. V. 627. P. 122225. https://doi.org/10.1016/j.ijpharm.2022.122225
Gao Y., Ji H., Peng L. et al. Development of PLGA-PEG-PLGA hydrogel delivery system for enhanced immunoreaction and efficacy of newcastle disease virus DNA vaccine // Molecules. 2020. V. 25. № 11. P. 2505. https://doi.org/10.3390/molecules25112505
Maeda T., Tanimoto K., Hotta A. Thermogelling nanocomposite hydrogel: PLGA molecular weight in P-LGA-b-PEG-b-PLGA affecting the thermogelling behavior // Macromolecular Chemistry and Physics. 2022. V. 223. № 1. P. 2100316. https://doi.org/10.1002/macp.202100316
Khorshid N.K., Zhu K., Knudsen K.D. et al. Novel structural changes during temperature-induced self-assembling and gelation of PLGA-PEG-PLGA triblock copolymer in aqueous solutions // Macromolecular Bioscience. 2016. V. 16. № 12. P. 1838–1852. https://doi.org/10.1002/mabi.201600277
Rahmani F., Atabaki R., Behrouzi S. et al. The recent advancement in the PLGA-based thermo-sensitive hydrogel for smart drug delivery // International Journal of Pharmaceutics. 2023. V. 631. P. 122484. https://doi.org/10.1016/j.ijpharm.2022.122484
Jin X., Fu Q., Gu Z. et al. Injectable corilagin/low molecular weight chitosan/PLGA-PEG-PLGA thermosensitive hydrogels for localized cancer therapy and promoting drug infiltration by modulation of tumor microenvironment // International Journal of Pharmaceutics. 2020. V. 589. P. 119772. https://doi.org/10.1016/j.ijpharm.2020.119772
Zhang L., Shen W., Luan J. et al. Sustained intravitreal delivery of dexamethasone using an injectable and biodegradable thermogel // Acta Biomaterialia. 2015. V. 23. P. 271–281. https://doi.org/10.1016/j.actbio.2015.05.005
Osorno L.L., Maldonado D.E., Whitener R.J. et al. Amphiphilic PLGA-PEG-PLGA triblock copolymer nanogels varying in gelation temperature and modulus for the extended and controlled release of hyaluronic acid // Journal of Applied Polymer Science. 2019. V. 137 № 25. P. 48678. https://doi.org/10.1002/app.48678
Chen X., Chen J., Li B. et al. PLGA-PEG-PLGA triblock copolymeric micelles as oral drug delivery system: In vitro drug release and in vivo pharmacokinetics assessment // Journal of Colloid and Interface Science. 2017. V. 490. P. 542–552. https://doi.org/10.1016/j.jcis.2016.11.089
Cao D., Zhang X., Akabar M. et al. Liposomal doxorubicin loaded PLGA-PEG-PLGA based thermogel for sustained local drug delivery for the treatment of breast cancer // Artificial Cells, Nanomedicine, and Biotechnology. 2019. V. 47. № 1. P. 181–191. https://doi.org/10.1080/21691401.2018.1548470
Wang P., Zhuo X., Chu W., Tang X. Exenatide-loaded microsphere/thermosensitive hydrogel long-acting delivery system with high drug bioactivity // International Journal of Pharmaceutics. 2017. V. 528. № 1–2. P. 62–75. https://doi.org/10.1016/j.ijpharm.2017.05.069
Yan Q., Xiao L.Q., Tan L. et al. Controlled release of simvastatin-loaded thermo-sensitive PLGA-PEG-P-LGA hydrogel for bone tissue regeneration: In vitro and in vivo characteristics // Journal of Biomedical Materials Research Part A. 2015. V. 103. № 11. P. 3580–3589. https://doi.org/10.1002/jbm.a.35499
Rong X., Ji Y., Zhu X. et al. Neuroprotective effect of insulin-loaded chitosan nanoparticles/PLGA-PEG-P-LGA hydrogel on diabetic retinopathy in rats // International Journal of Nanomedicine. 2019. V. 14. P. 45–55. https://doi.org/10.2147/IJN.S184574
Дополнительные материалы отсутствуют.
Инструменты
Коллоидный журнал