Теплоэнергетика, 2023, № 8, стр. 3-26
Перспективы использования двумерных наноматериалов в энергетических технологиях (обзор)
А. С. Дмитриев a, b, *, А. В. Клименко c, **
a Национальный исследовательский университет “Московский энергетический институт”
111250 Москва, Красноказарменная ул., д. 14, стр. 1, Россия
b Институт графена
119019 Москва, Никитский бульв., д. 12, Россия
c Национальный исследовательский технологический университет
“Московский институт стали и сплавов” (МИСиС)
119049 Москва, Ленинский просп., д. 4, Россия
* E-mail: asdmitriev@mail.ru
** E-mail: klimenkoav@bk.ru
Поступила в редакцию 23.01.2023
После доработки 15.02.2023
Принята к публикации 01.03.2023
Аннотация
Рассмотрены перспективы применения новых двумерных наноматериалов (2D-материалов) для интенсификации процессов тепломассообмена в энергетическом оборудовании. Представлены основные типы 2D-материалов, описаны их физико-технологические свойства и уникальные характеристики. Изучены отдельные технологические приемы изготовления двумерных материалов и создаваемых пленок, композитов, наножидкостей для различных энергетических приложений. Отдельное внимание уделено материалам на основе однослойного и многослойного графена, характеристикам материалов на базе графеновых компонентов, их физико-химическим и другим параметрам. Описаны эффекты, которые наблюдаются при использовании наножидкостей в качестве теплоносителей и при нанесении покрытий на основе 2D-материалов на теплопередающие поверхности при различных процессах теплообмена: однофазной конвекции, испарении, кипении, конденсации. Показано, что во всех случаях существенно повышается эффективность теплопереноса. Приведены конкретные примеры применения 2D-материалов в тепловых трубах и термосифонах, описаны способы улучшения характеристик этих устройств. Рассмотрены некоторые математические и физические модели функционирования двумерных материалов в энергетике. Особое внимание уделено особенностям механизмов теплопереноса, испарения, кипения и конденсации в энергетических системах. Однако указано, что имеются определенные трудности при выборе двумерных материалов для использования их в энергетике. Сделан вывод, что благодаря дополнительным исследованиям и активному применению 2D-материалов открываются беспрецедентные возможности для развития перспективных энергетических, строительных, электронных и других технологий, а также создания материалов следующего поколения с уникальными механическими, оптическими, электромагнитными и тепловыми свойствами.
Развитие энергетических технологий всегда было связано с появлением новых материалов. Так, эффективность традиционной тепловой энергетики за последние 100 лет возросла в 4 раза во многом благодаря созданию и применению металлов, имеющих требуемые прочностные характеристики при высоких температурах. В последние годы, однако, в традиционной и альтернативной (безуглеродной) энергетике возникли новые задачи в связи с жесткими требованиями к физико-химическим и механическим свойствам функциональных материалов [1–4]. Развернуты широкомасштабные исследования по разработке таких материалов. Их обзор представлен в [5–11]. Особое место занимают наноматериалы, которые обладают уникальными свойствами и потому находят широкое применение в самых разных областях [12–26]. Таким материалам посвящено значительное число исследований, в том числе их использованию в энергетических технологиях [19–26].
Тема данного обзора довольно узкая – применение двумерных наноматериалов и конструкций из них. Главной особенностью 2D-материалов является их толщина, которая не превышает толщины атомарного слоя (не более 1 нм). Все атомы такого “листового” материала находятся в поверхностном слое, что и определяет уникальность его свойств (исключительно высокие тепло- и электропроводность, прочность при растяжении на разрыв). Важно отметить, что свободные связи атомов позволяют модифицировать свойства материала путем легирования атомами других элементов.
На рис. 1 представлены семейства разработанных двумерных материалов.
Родоначальник подобных материалов – хорошо известный графен, который в настоящее время широко применяется в различных технологиях, в том числе и энергетических. С момента открытия графена технологии на его основе стали самой быстрорастущей отраслью науки в мире (до 38% роста в год). По оценкам, рынок графена (разработка, производство и продажа) к 2030 г. составит не менее 400 млрд дол. Помимо графена и его вариаций [многослойных графеновых нанохлопьев (graphene nanoflakes – GNF) и модифицированных вариантов графена], в последние годы появились новые двумерные материалы – объемные слоистые структуры, из которых можно выделить отдельные слои толщиной в несколько атомов: дихалькогениды переходных металлов (transition metal dichalcogenides – TMD) [6, 14], карбиды, нитриды и карбонитриды переходных металлов (максены) [16] и оксиды переходных металлов (transition metal oxides – TMO) [17, 18]. Такие материалы образуют отдельный класс двумерных материалов – 2D-гетероструктуры.
Двумерные материалы к настоящему времени довольно подробно изучены и используются во многих устройствах накопления и преобразования энергии [16, 19–30], в том числе в фотоэлектрических и солнечных преобразователях энергии [31, 32] и пьезоэлектрических и термоэлектрических устройствах [33–37].
Вместе с тем, 2D-материалы обладают свойствами, которые могут радикально изменить отношение к некоторым технологиям в существующих секторах энергетики:
в тепловой и атомной энергетике – создание с помощью 2D-материалов эффективных тепломассообменных поверхностей, используемых при испарении, кипении и конденсации жидкостей, в системах очистки и обессоливания воды и т.п. [11–13];
в гидроэнергетике – улучшение механических характеристик конструкций, в том числе бетонных сооружений;
в установках на основе возобновляемых источников энергии – повышение механической прочности лопастей ветровых электростанций и снижение издаваемого ими шума, улучшение эрозионных характеристик фотоэлектрических панелей и создание супергидрофобных поверхностей для них и др.
Возможные направления использования 2D‑материалов в энергетике представлены на рис. 2.
В настоящем обзоре основное внимание будет уделено возможности применения 2D-наноматериалов для интенсификации процессов тепломассообмена, широко распространенных в теплоэнергетике и теплотехнике: однофазной конвекции, кипения, конденсации, испарения.
ФИЗИКО-ХИМИЧЕСКИЕ СВОЙСТВА ГРАФЕНА И КОМПОЗИЦИЙ НА ЕГО ОСНОВЕ
Свойства большинства 2D-материалов описаны в работах [19–26]. В данной же статье внимание уделено главным образом графену и его производным применительно к процессам переноса тепла (рис. 3).
К графеновым материалам относят не только сам графен, но и его оксид (GO), восстановленный оксид графена (rGO), а также гетероатомный, функционализированный и трехмерный (3D) графен (см. рис. 3). Свойства графена одноатомной толщины – однослойного графена (single graphene layer – SGL) – сохраняются и в многослойном графене (few graphene layers – FGL) при числе слоев N, не превышающем 10. При N > 10 многослойный графен приобретает свойства обычного графита [19, 26]. Помимо этого, существуют так называемая графеновая пена и другие аналоги трехмерных графеновых структур (рис. 4).
Материалы на основе графена демонстрируют уникальные физические и химические свойства, такие как большая удельная площадь поверхности, отличные электропроводность и теплопроводность, высокая механическая прочность и хорошая химическая стабильность [9–13].
Графен имеет значительную теоретическую площадь поверхности (до 2630 м2/г), что примерно на два порядка больше, чем у графитового порошка (около 10 м2/г) [10]. Двумерные материалы на основе графена с большой площадью поверхности могут иметь повышенную адсорбционную способность к реагентам вследствие сильного взаимодействия с π-электронами, что позволяет использовать их в качестве катализаторов [11]. Графен – полупроводник с нулевой запрещенной зоной, с высокими подвижностью носителей [приблизительно 10 000 см2/(В · с)] и скоростью электронов (примерно 106 м/с) из-за перекрытия валентной зоны и зоны проводимости. Подвижность носителей в графене меньше подвержена влиянию температуры, поэтому их сверхвысокая мобильность достигается уже при комнатной температуре.
Большое значение для энергетики и электроники имеет очень высокая теплопроводность однослойного графена – около 5000 Вт/(м · К), по некоторым данным – до 7500 Вт /(м · К) (для сравнения, теплопроводность меди на порядок ниже). Это позволяет применять материалы на базе графена в термоменеджменте энергетических и электронных устройств [9, 37], что особенно важно для систем, соответствующих требованиям строгого регулирования температуры, в частности при реакциях, в которых проявляется сильная эндо- или экзотермичность [9].
Графен обладает хорошими механическими свойствами и высокой термостойкостью. Бездефектный графен имеет большой модуль Юнга (приблизительно 1.0 ТПа) и значительный критический уровень разрушения – предельная прочность равна примерно 130 ГПа [9]. Графен устойчив к окислению вплоть до 300°C [11].
Для большинства приложений в энергетических технологиях требуется так называемая функционализация графена и других 2D-материалов, например, гетероатомное легирование. Благодаря гибкой углеродной основе графена появляются фактически безграничные возможности для его модификации и функционализации. Допирование гетероатомами (например, азота, бора, серы, галогенов, благородных или переходных металлов) как базисной плоскости, так и реактивных краев графена позволяет соответствующим образом “настраивать” его теплофизические, электрохимические и каталитические свойства.
Еще одним классом графеновых материалов, весьма перспективных для использования в энергетике, являются графеновые нанокомпозиты и наножидкости [9, 37]. Первые – это структуры, состоящие из матричного материала (например, металла, керамики, полимеров и т.д.) и графеновых нанохлопьев или хлопьев (двумерных графеновых структур из однослойного или многослойного графена), специально “сморщенных” или предварительно функционализированных. На рис. 5 представлены некоторые варианты таких материалов. В настоящее время активно исследуется возможность использовать их в качестве термостабилизаторов в электронных и энергетических устройствах, а также в солнечной теплоэнергетике [6, 37–48].
Рис. 5.
Графен с добавлением жидкого металла (галлистан, 6%) (а), графеновые хлопья с наночастицами нитрида бора (4%) (б) и наночастицами нитрида алюминия (4%) (в)
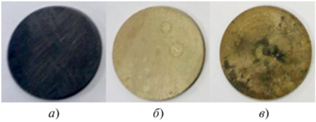
Различные типы наножидкостей позволяют существенно интенсифицировать процесс солнечной генерации пара и тем самым открывают новые перспективы создания электростанций с паросиловым циклом [6].
ПРИМЕНЕНИЕ ДВУМЕРНЫХ МАТЕРИАЛОВ В ТЕПЛОЭНЕРГЕТИКЕ
Использование наноматериалов в тепловой и атомной энергетике связано прежде всего с возможностью интенсификации процессов тепломассообмена, влияющих на эффективность работы оборудования.
Существуют различные способы применения графеновых материалов:
в качестве теплоносителя графеновых наножидкостей [базовая жидкость + графеновые добавки в виде однослойного или многослойного графена различной геометрии (пластины, хлопья, “сморщенные” структуры) и с латеральными размерами в диапазоне 10–100 мкм];
путем нанесения на поверхность теплообмена графеновых пленок (сплошных или пористых);
в виде графеновых мембран (наноструктуры с ламинированным расположением графеновых хлопьев – регулярные или хаотические, с закрытой или открытой лабиринтовой структурой) или графеновых объемных материалов (графеновые композиты, гибридные графеновые композиты, графеновые пены или нанопористые нерегулярные структуры) в теплопередающих устройствах.
Теплоотдача к графеновым наножидкостям
Значительное число исследований посвящено графеновым наножидкостям и их использованию в различном теплообменном оборудовании. Были изучены как процессы теплопроводности в подобных наножидкостях, так и ламинарное и турбулентное течения, а также естественная и вынужденная конвекция [36, 38–42, 48–60]. На рис. 6 приведены относительные свойства графеновой наножидкости к базовой жидкости [44].
Рис. 6.
Зависимость относительных свойств наножидкости Р в сравнении со свойствами базовой жидкости от концентрации графена φGNF. 1 – теплопроводность; 2 – вязкость; 3 – плотность; 4 – коэффициент поверхностного натяжения
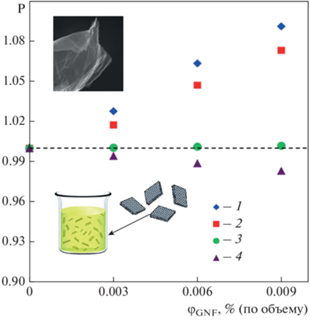
В работе [36] представлено экспериментальное исследование теплоотдачи при однофазной вынужденной конвекции водных суспензий наночастиц оксида графена. Процесс был рассмотрен в диапазоне объемных концентраций графеновых наночастиц [от 0.05 до 0.20% (по объему)] при течении в стальной трубе (длиной 2000 мм, внутренним диаметром 3.96 мм и толщиной стенки 0.17 мм) при различных тепловых нагрузках на стенки. Значения числа Рейнольдса Re в опытах изменялись в пределах от 3 × 103 до 1.1 × 104 (фактически турбулентные режимы течения). В результате проведенного исследования было установлено, что:
вязкость наножидкости на основе оксида графена становится больше с ростом объемной доли наночастиц и ослабевает при повышении температуры наножидкости (рис. 7, а);
Рис. 7.
Зависимость коэффициента динамической вязкости μ наножидкости на основе оксида графена от температуры t и концентрации наночастиц С (а) и зависимость числа Nu от числа Re (б). 1 – чистая вода; С, % (по объему): 2 – 0.05; 3 – 0.10; 4 – 0.15; 5 – 0.20; 6 – модель Gnielinski [52]
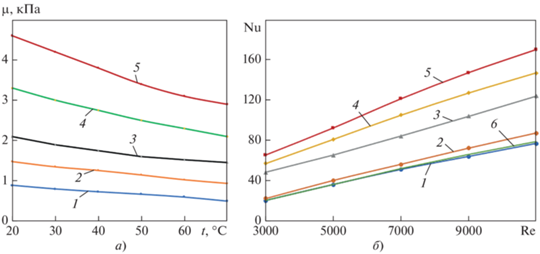
потери давления в наножидкости увеличиваются незначительно по сравнению с чистой водой (от 3 до 7% в зависимости от концентрации оксида графена);
как и в случае с чистой водой, для всех объемных концентраций происходит рост числа Нуссельта Nu с увеличением числа Рейнольдса (расчет по методу [52] согласуется с экспериментальными данными для турбулентных течений);
теплоотдача при течении в круглой трубе наножидкости оказывается выше по сравнению с чистой водой (рис. 7, б). При этом в исследованном диапазоне наблюдается увеличение теплоотдачи при повышении концентрации наночастиц (см. таблицу). Таким образом, при развитом турбулентном течении интенсификация теплоотдачи вследствие добавления наночастиц значительно превышает рост гидравлического сопротивления.
Увеличение числа Нуссельта при повышении концентрации наночастиц оксида графена в водной суспензии по сравнению с чистой водой, разы
Число Re | Концентрация наночастиц, % (по объему) | |
---|---|---|
0.05 | 0.20 | |
3000 | 1.10 | 3.25 |
11 000 | 1.13 | 2.21 |
В [40] были проведены численное и экспериментальное исследования теплогидравлических характеристик при течении воды с добавлением нанопластинок графена и наночастиц диоксида титана в горизонтальной трубе диаметром 17 мм, длиной 1100 мм, с внешним обогревом тепловым потоком 50 кВт/м2. Опыты были поставлены на жидкости с различными массовыми долями нанокомпонентов (0.50, 0.75 и 1.00%) при ее турбулентном течении (Re = 2000–7300).
Теплопроводность наножидкостей вычислялась по соотношению для эффективной теплопроводности (аналог модели Максвелла [9])
Теплопроводность графеновой наножидкости на водной основе [содержание графена 0.10% (по массе)] увеличилась примерно на 17% по сравнению с теплопроводностью деионизированной воды при температуре наножидкости 25°C и почти на 29% (по массе) при 45°С (температура воды равна 25 и 45°C соответственно).
Таким образом, для фиксированной концентрации наночастиц рост теплопроводности зависит от температуры для указанного рабочего диапазона. Следует отметить, что вязкость графеновой наножидкости повышается на 175% при ее температуре 20°C и содержании графена 0.10% (по массе) и снижается на 25% при изменении температуры от 20 до 50°C.
Зависимости числа Нуссельта и перепада давления от числа Рейнольдса представлены на рис. 8. Как и в работе [36], добавление наночастиц привело к росту теплоотдачи по сравнению с чистой водой. Так, число Нуссельта при концентрации графеновых нанопластинок 1% (по массе) увеличилось в 4.8 раза для Re = 5000 и в 4.2 раза для Re = 7000. При этом гидравлическое сопротивление повысилось существенно меньше – до 30%. Таким образом, наножидкости вновь продемонстрировали способность преодолевать аналогию Рейнольдса, тем самым давая исследователям возможность получать более значительное увеличение теплоотдачи по сравнению с ростом гидравлического сопротивления.
Рис. 8.
Зависимость числа Нуссельта Nu (а) и перепада давления Δр (б) от числа Рейнольдса Re. 1 – чистая вода; φGNF, % (по массе): 2 – 0.50; 3 – 0.75; 4 – 1.0; 5 – ${{\varphi }_{{{\text{Ti}}{{{\text{O}}}_{{\text{2}}}}}}}$ = 1% (по массе)
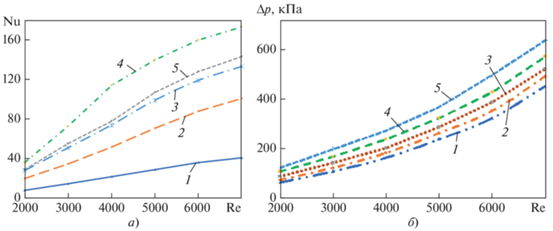
Процессы, происходящие в графеновых наножидкостях, связаны с особенностями самих графеновых нанохлопьев: во-первых, последние испытывают в жидкости эффект проскальзывания (касательное напряжение близко к нулю); во-вторых, масса графеновых нанохлопьев чрезвычайно мала (в несколько раз меньше, чем даже наночастиц), что позволяет легко переносить их с базовой жидкостью. Помимо этого, теплопроводность графеновых нанохлопьев в несколько раз выше, чем у любых указанных ранее наночастиц.
Если сопоставить эффекты, оказываемые на теплоотдачу, при добавлении нанопластинок графена и наночастиц диоксида титана при их одинаковой концентрации 1%, то станет ясно, что во всем исследованном диапазоне чисел Re явное преимущество имеет графен. Наибольшее полученное число Нуссельта (при Re = 7000) составляет приблизительно 133 для наножидкости диоксид титана + вода и около 173 для наножидкости графеновые нанопластинки + вода. К тому же оказывается, что коэффициент трения последней ниже, чем у наножидкости диоксид титана + вода, при одинаковых Re. Можно сделать вывод, что графеновые жидкости эффективнее при всех числах Рейнольдса (как по теплопередаче, так и по гидравлическому сопротивлению).
Согласно выводам авторов работы [53], в которой проводилось численное моделирование турбулентного течения воды с добавками наночастиц в теплообменнике (типа труба в трубе, диаметром 8 мм, длиной до 6000 мм) в температурном диапазоне наножидкости от 20 до 70°С, концентрация наночастиц графена составляла 0.025, 0.050, 0.075 и 0.100% (по объему) при варьировании числа Рейнольдса от 5 × 103 до 36.5 × 103. Результаты расчетов хорошо согласуются с экспериментальными данными, опубликованными, например, в [53, 54]. Отмечено, что наножидкость графен – вода имеет потенциальные преимущества перед другими наножидкостями и чистыми рабочими жидкостями (например, водой или этиленгликолем). Несмотря на увеличение гидравлических потерь (до 33% в наножидкостях с высокой концентрацией графена – до 0.1%), опережающий рост коэффициента теплоотдачи позволяет существенно улучшить параметры теплообменников, в частности заметно снизить эксплуатационные расходы на теплообменные системы.
Теплофизические свойства графеновых наножидкостей в двухфазном термосифоне были изучены при различных мощностях нагрева, температурах и углах наклона оси термосифона к горизонту [36, 38, 43]. Углы наклона изменялись от 0 до 30°. Размеры термосифона: испаритель – 50 см, конденсатор – 40 см. Тепловая нагрузка в-арьировалась от 20 до 70 Вт. Обнаружено, что при 45°C теплопроводность графеновой наножидкости на 29% выше, чем у деионизированной воды. Вязкость графеновой наножидкости повышается с ростом концентрации наночастиц графена и уменьшается с увеличением температуры. Тепловое сопротивление термосифона (отношение подводимой мощности к мощности испарения) снижается при повышении потребляемой мощности независимо от угла наклона его оси. Проведенные исследования показывают, что термосифон, в котором применяется вода с наночастицами Al2O3, CuO и TiO2, уступает по базовым параметрам аналогичному термосифону с графеновой наножидкостью вместо воды. Однако проведенных исследований недостаточно, чтобы установить преимущества таких наножидкостей перед другими жидкостями.
Механизм интенсификации тепломассообмена при пузырьковом кипении при использовании графеновых наножидкостей или графеновых подложек (в форме микропленок графена или специальных графеновых вставок) в настоящее время вполне освоен. Главное, что влияет на рост критического теплового потока (КТП) и коэффициента теплоотдачи, – высокая теплопроводность графена и формирование на самой поверхности или вблизи нее нанопористого слоя, способствующего генерации пара внутри нанопор. Это приводит к усилению генерации пузырей при кипении, движению жидкости к основанию пузыря и эффективному испарению около “сухих” пятен, как это показано на рис. 9 [43]. Здесь представлена картина осаждения графеновых нанохлопьев на поверхность теплообмена, что приводит к образованию мест с эффективной генерацией микропузырей и их быстрому росту из-за нанопористости слоя и его высокой теплопроводности.
Рис. 9.
Формирование слоя графеновых отложений из поверхности нагрева (а), образование нанопористого микрослоя из графена (б), генерация микропузырей внутри нанопористого слоя на поверхности нагрева (в), зависимость теплового потока q от температуры t (кривая кипения) (г). 1 – графеновая подложка или графеновая наножидкость; 2 – без графена; 3 – КТП
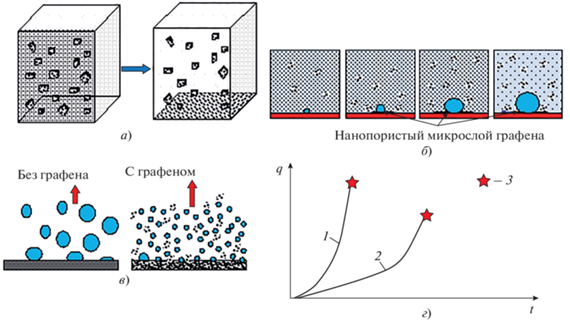
Особое внимание в атомной энергетике уделяется в настоящее время внешнему охлаждению корпуса реактора [35, 50]. Повышению критического теплового потока для обеспечения более высокого уровня безопасности атомных реакторов посвящено исследование [49], в котором оксид графена наносили на теплоотдающую поверхность, моделируя корпус реактора высокого давления. В качестве охлаждающей жидкости использовали хладагент R-123 с добавленными в него хлопьями оксида графена. В экспериментах применяли наножидкости с различной концентрацией этих частиц (хлопьев): 0.01, 0.03 и 0.05% (по объему). Показано, что критический тепловой поток возрастает по сравнению с обычным теплоносителем и не покрытой графеном поверхностью на 38% при массовой доле оксида графена 0.05%. Отмечено также интересное поведение пузырей при пузырьковом режиме кипения – так называемые пузырьковые волны вблизи КТП: поскольку нагреватель представлял собой полусферическое тело, при повышении тепловой нагрузки области активного пузырькового кипения волнами “сползали” в нижнюю часть нагревателя, интенсифицируя процесс передачи тепла.
Процессы теплоотдачи на поверхностях с покрытиями из 2D-материалов
Для термостабилизации электронного и энергетического оборудования необходимо решать задачи, возникающие при капельно-струйном и испарительном его охлаждении в широком диапазоне температур (от комнатной до температуры выше температуры пленочного кипения) [46, 50, 56, 58–60].
Испарение капель при умеренной температуре графеновой подложки. В [56] изучался процесс испарения капли воды, помещенной на горячую медную поверхность с графеновым покрытием, температура которой не превышала 100°С, что обеспечивало прямой контакт между каплей и поверхностью. Для нанесения покрытий были использованы два метода модификации поверхности – химическое осаждение из паровой фазы (chemical vapor deposition – CVD) и золь-гельный метод (рис. 10).
Рис. 10.
Вид гладкой медной поверхности (а) и поверхностей с покрытием, нанесенным графеновым методом CVD (б) и золь-гельным методом (в)
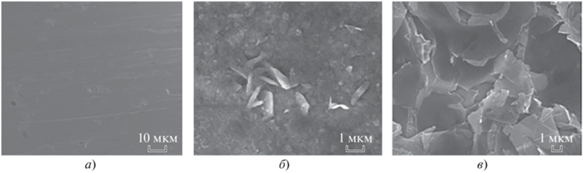
Схема эксперимента по исследованию тепломассообмена при испарении капель на модифицированных графеном поверхностях представлена на рис. 11. На поверхность с помощью дозатора помещалась капля воды, которая испарялась из-за подводимого от блок-нагревателя тепла. Размеры капель варьировались от 2 до 4 мм. Измерялась температура подложки под каплей и вне ее термопарами (рис. 11). Методом оптической фиксации объема капель были определены также относительная тепловая мощность, отводимая от гладкой медной поверхности, и тепловая мощность, отводимая при испарении капель на различных поверхностях, в зависимости от времени. Результаты измерения тепловых мощностей приведены на рис. 12.
Рис. 11.
Схема установки по исследованию тепломассообмена при испарении капель на модифицированных графеном поверхностях. 1 – блок-держатель; 2 – дозатор; 3 – капля; 4 – поверхность с покрытием; 5 – термопара; 6 – блок-нагреватель; 7 – источник питания
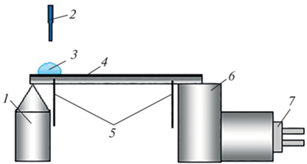
Рис. 12.
Зависимость отводимой от поверхности относительной тепловой мощности $\bar {Q}$ от температуры t (а) и отводимой тепловой мощности при испарении капель Q при температуре поверхности 85°С от времени τ (б). 1 – медная поверхность; 2 – графеновое покрытие (метод CVD); 3 – графеновое покрытие (золь-гельный метод)
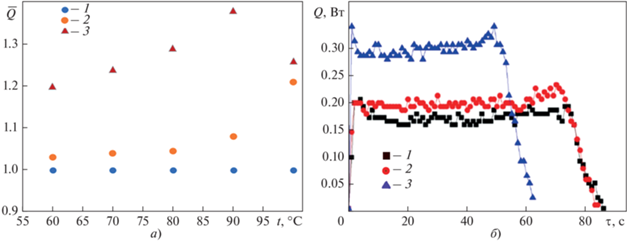
Опыты показали, что, по сравнению с гладкой медной поверхностью, поверхность с графеновым покрытием обеспечивает более интенсивное испарение. Стоит отметить, что с покрытий, полученных золь-гельным методом, происходило более эффективное испарение, что связано, в основном, с изменением смачивания поверхности.
Испарение капель и эффект Лейденфроста. Процессы испарения капель на графеновых поверхностях в широком диапазоне температур (вплоть до температуры пленочного кипения и выше) и эффект Лейденфроста рассмотрены в цикле публикаций [61–65]. В отличие от других исследователей, авторы [61–65] работали с чистыми графеновыми нанохлопьями, полученными методом их суперкавитационной эксфолиации в дистиллированной воде, поэтому нанохлопья не были окислены. В процессе изучения тепломассообмена при испарении капель различных жидкостей (воды, спирта, этиленгликоля и т.п.) хлопья несколько окислялись, но, как показала рамановская спектроскопия, только по границам, что оставляло их фактически не окисленными.
Подготовленные образцы представляли собой поверхности с плотной упаковкой графеновых нанохлопьев (свободных либо связанных внутри медными микросетками) при давлении 20–50 МПа (рис. 13). Были изучены контактные углы при смачивании каплями графеновых подложек и скорости испарения капель, размер которых в-арьировался от 1 до 4 мм (см. рис. 13). Следует отметить, что изменение контактных углов весьма существенно зависит от температуры поверхности, на которую падают капли. Угол резко уменьшается с ростом температуры, что можно наблюдать главным образом при режиме постоянной контактной линии. Такая ситуация довольно необычна, поскольку при использовании других материалов (в частности, металлических мезо- и наноструктур) происходит смена режима постоянного контактного угла на режим постоянства контактной линии при увеличении температуры. Было показано также, что до температуры пленочного кипения (температура Лейденфроста) капля испаряется примерно с одинаковой скоростью со всех подложек. Вместе с тем, температура Лейденфроста графеновых подложек, особенно с сеткой, оказалась заметно выше, чем у алюминиевых подложек. Этот эффект, по-видимому, связан с уникально быстрым проникновением воды внутрь графена, что позволяет пару выходить через наноструктуру вне области под каплей, препятствуя образованию непрерывного слоя пара, необходимого для левитации капель.
Рис. 13.
Испарение капель на графеновых подложках, заделанных в медные сетки, при начальном диаметре капель 3 мм. а – поверхность испарения; б – зависимость краевого угла смачивания θ от времени испарения τи; в – зависимость времени испарения капель от температуры поверхности. t, °С: 1 – 20; 2 – 30; 3 – 40; 4 – 50; 5 – 60; 6 – 70; 7 – 80; 8 – 90; 9 – чистый алюминий; 10 – графеновое покрытие; 11 – графеновое покрытие с сеткой
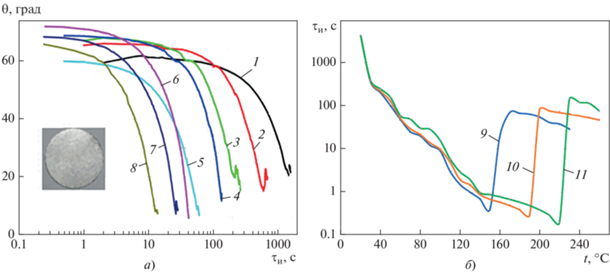
В работах [62–64] были измерены скорости испарения чистой воды с поверхности графенового покрытия и графенового покрытия на мезопористой подложке из алюминия. На рис. 14 представлены указанные типы графеновых структур (в начальный момент вся вода находилась внутри графенового слоя). Отмечено, что скорость испарения со свободной поверхности воды в несколько раз ниже, чем с поверхности графенового слоя. Разница может составлять от 2.5 до 8 раз при тепловых потоках в диапазоне от 50 до 180 кВт/м2 (вода поступает в слой из-за сильного впитывания капиллярами через нижнюю часть графенового слоя) [65, 66].
Рис. 14.
Графеновые структуры для исследования скоростей испарения. а – графеновый слой, насыщенный водой; б – мезоструктура графенового слоя; в – графен на мезопористом алюминии (поры 0.2–5.0 мкм); г – структура графенового слоя (поры 25–150 нм)
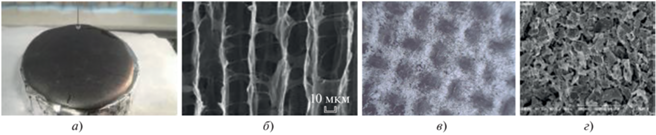
Наличие мезопористого слоя алюминия позволяет увеличить скорость испарения еще примерно на 11–14%. При отсутствии мезопористого покрытия скорость испарения остается высокой, но все-таки меньше, чем с использованием нанохлопьев графена без подложки.
Таким образом, можно утверждать, что чистый графен отлично впитывает и испаряет воду, что очень важно при реализации технологий получения чистой воды и обессоливания. Результаты работы [66] подтверждаются и данными других исследователей [67].
Кипение на модифицированных поверхностях. Кипение на наноструктурированных поверхностях исследуется довольно давно [9]. Установлены многие особенности коэффициентов теплоотдачи, критических тепловых потоков и других параметров кипения (см., например, [46, 48–50, 56, 58]). Наиболее впечатляющие результаты получены с использованием различных материалов на основе графена и иных двумерных материалов [9]. Для создания поверхностных покрытий графен интересен прежде всего из-за его превосходной теплопроводности. Помимо этого, графен и его оксиды обладают уникальным свойством образовывать стабильные нанопористые структуры, которые, согласно существующим представлениям, могут заметно влиять на механизмы парообразования при кипении [9, 48]. В частности, в работе [46] приведены экспериментальные данные об исключительно высоких коэффициенте теплоотдачи и критическом тепловом потоке qс при кипении воды, этиленгликоля и других жидкостей в большом объеме при атмосферном давлении на поверхности медных образцов диаметром 2.5–5.0 см, имеющих графеновое покрытие, декорированное медными микрочастицами (рис. 15).
Рис. 15.
Зависимость теплового потока q от температурного напора Δt (а), коэффициента теплопередачи α от теплового потока (б) и морфология графеновых структур (в) при кипении воды на поверхностях с графеновыми покрытиями, декорированными медными частицами. 1 – гладкая медная поверхность; 2 – электроосаждение наноколлоидного раствора графеновых хлопьев (2%) на поверхности меди; 3 – декорированная медными частицами (20 мкм) графеновая поверхность (2%) после спекания; 4 – декорированная медными частицами (20 мкм) графеновая поверхность (3%) после спекания. qc, Вт/см2: 1 – 125; 2 – 239; 3 – 286; 4 – 289
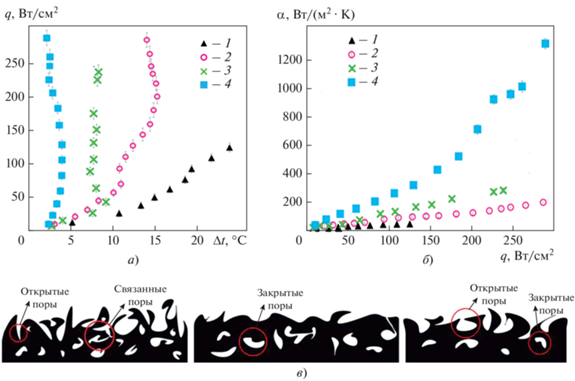
Важно отметить, что на различных поверхностях, исследованных в работе [46], пузыри имеют разную скорость роста, как это показано на рис. 16. В частности, наличие многомасштабной морфологии поверхности (мезо- и наноразмерные поры, открытые или закрытые лабиринтовые структуры, “регулярное” расположение нанопор и наноканалов или их хаотичное распределение, особенности поведения жидкости в нанопоровом пространстве ввиду нетривиальной капиллярной термогидродинамики [66]) значительно влияет на все стадии процесса кипения – от зарождения, роста пузырей и их отрыва до явлений подтекания жидкости к “сухим” пятнам и всплытия пузырей. Нанопористая структура графеновых покрытий позволяет капиллярным силам стягивать воду внутри покрытий, что обеспечивает существенно более эффективное образование паровой фазы и генерацию пара с заметно большей скоростью.
Рис. 16.
Зависимость диаметра пузырей d от времени при кипении воды на различных поверхностях при атмосферном давлении. Обозначения см. рис. 15
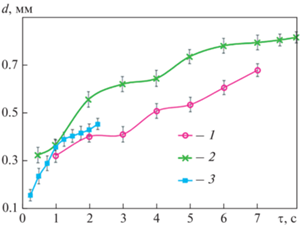
По представленной в работе [46] новой технологии получения высокоактивных, функциональных и настраиваемых иерархически пористых поверхностей с графен-медным покрытием в экспериментах по пузырьковому кипению воды при атмосферном давлении был достигнут высокий критический тепловой поток – около 289 Вт/см2 при перегреве стенки Δt всего на 2.2°C для 3% (по массе) чистых графеновых нанохлопьев, декорированнных медными частицами диаметром около 20 мкм. Более высокая, чем у меди, теплопроводность покрытия способствовала заметному возрастанию критического теплового потока, а нанопористость поверхности приводила к повышенной термической активности (или скорости генерации пузырей), по сравнению с гладкими или даже шероховатыми поверхностями. Термическая активность определялась толщиной слоя покрытия и его теплофизическими свойствами. При ее увеличении развитие локальной горячей точки в необратимую “сухую” точку могло быть подавлено при пузырьковом кипении вблизи критического теплового потока.
Работа [56] посвящена созданию оптимальной поверхности кипения на основе покрытий из оксида графена. Такая поверхность обеспечивает существенную интенсификацию процесса кипения и увеличение теплоотдачи: для воды критический тепловой поток qc ≈ 261 Вт/см2, коэффициент теплоотдачи равен приблизительно 9.1 Вт/(см2 ⋅ К). Эти показатели являются одними из самых высоких из полученных для теплопередачи при кипении в большом объеме на плоских модифицированных поверхностях.
Такие параметры кипения авторы объясняют формированием тесно связанной с подложкой ламинированной пленки оксида графена умеренной толщины, обладающей высокой теплопроводностью, что облегчает быстрое охлаждение и повторное смачивание локальных горячих/сухих областей, а также зарождение пузырей и тем самым задерживает возникновение пленочного режима кипения. Следует отметить, что покрытие обладает хорошей стойкостью. На рис. 17 представлена зависимость числа центров парообразования от теплового потока при кипении при оптимальной морфологии поверхности кипения (время отложения оксида графена на медной поверхности составляет около 2.5 ч). Хорошо видно, что число центров парообразования для покрытой графеном поверхности при любых тепловых нагрузках в несколько раз выше, чем для гладкой медной поверхности.
Рис. 17.
Зависимость числа центров парообразования n от теплового потока при кипении. 1 – гладкая медная поверхность; 2 – поверхность, покрытая графеном
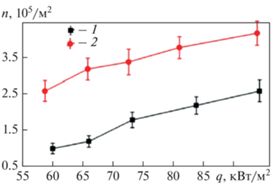
Как показано в [57], эффективность пузырькового кипения можно значительно повысить, используя наноструктурированную поверхность графена. Смачиваемость поверхности, покрытой графеновыми нанопластинками (graphene nanoplates – GNP), можно регулировать с помощью процесса термического отверждения. Неотвержденная поверхность GNP гидрофобна по своей природе, в то время как поверхность отвержденных GNP проявляет гидрофильные свойства: она работает лучше, чем неотвержденная, в режиме пузырькового кипения. В конечном итоге, благодаря сверхбыстрой водопроницаемости отвержденных GNP повышается эффективность пузырькового кипения, что приводит к максимальному росту коэффициента теплопередачи при кипении – на 151%, а массового расхода пара – на 154%. Способность к более эффективному кипению неотвержденной поверхности GNP также повышается, несмотря на ее гидрофобность. Следовательно, интенсификация кипения связана не только со смачиваемостью поверхности, но и с эффектом сверхбыстрого проникновения воды в нанопористую структуру. Беспрепятственный перенос воды в сети наноканалов GNP способствует значительному поглощению скрытой теплоты испарения молекулами воды, что приводит к существенному увеличению зародышеобразования, росту и уходу пузырей пара.
Конденсация. Режим капельной конденсации, возникающий тогда, когда образующийся конденсат не смачивает поверхность теплообмена, значительно превосходит по своей интенсивности режим пленочной конденсации, при котором наблюдается смачиваемость поверхности. При использовании гидрофобных покрытий для развития капельной конденсации предполагается, что покрытие должно обладать износостойкостью, химической стабильностью и низким термическим сопротивлением. Этим требованиям наилучшим образом соответствуют графеновые покрытия теплообменной поверхности [45, 47].
В работе [47] показано, что эффективность сверхтонких масштабируемых графеновых покрытий, получаемых методом химического осаждения из паровой фазы (CVD) при низком и атмосферном давлениях, весьма высока в режиме капельной конденсации.
На рис. 18 приведены полученные с помощью электронной микроскопии изображения гладкой (см. рис. 18, а) и покрытой графеновым микрослоем (см. рис. 18, б) поверхности медной трубы (диаметром 8 мм и длиной 600 мм), на которой изучался процесс конденсации перегретого пара. Здесь же представлены процесс пленочной конденсации водяного пара температурой 100°С на поверхности из чистой меди (см. рис. 18, в) и капельная конденсация на поверхности с графеновым покрытием при тех же условиях (см. рис. 18, г).
Рис. 18.
Вид гладкой (а) и покрытой графеновым микрослоем (б) поверхности медной трубы и процессов конденсации водяного пара на поверхности из чистой меди (в) и на поверхности с графеновым покрытием (г)
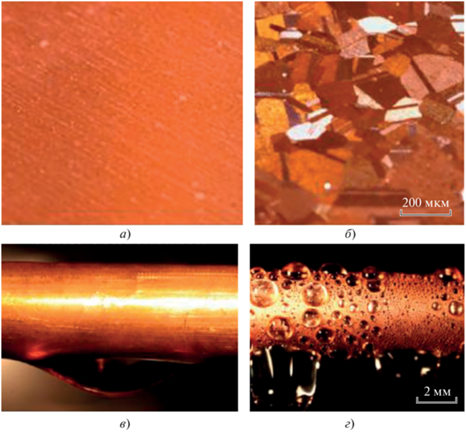
На рис. 19 показана зависимость теплового потока от перепада температур на поверхности трубы из чистой меди и меди, поверхность которой модифицирована графеновым микрослоем (химическим осаждением из паровой фазы при низком и нормальном давлениях).
Рис. 19.
Зависимость теплового потока от перепада температур Δt при конденсации воды на медной поверхности, модифицированной графеновым микрослоем (химическим осаждением из паровой фазы) при низком (1) и нормальном (2) давлении и на поверхности из чистой меди (3)
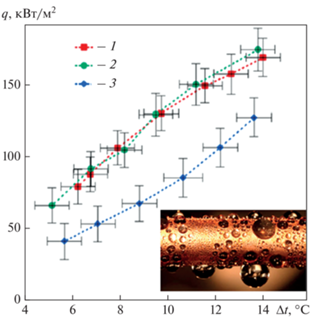
Коэффициент теплоотдачи при пленочной конденсации, как отмечают авторы [47], для медных труб с графеновым покрытием (CVD низкого давления и CVD атмосферного давления) составляет приблизительно 60 ± 20 кВт/(м2 ⋅ К), что в 4 раза больше, чем для пленочной конденсации на чистой меди [примерно 15 ± 9 кВт/(м2 ⋅ К)]. Стоит отметить, что коэффициент теплоотдачи при капельной конденсации уменьшается при малых недогревах, так как межфазный теплообмен дает основной вклад в термическое сопротивление; коэффициент теплоотдачи при пленочной конденсации возрастает при малых недогревах по мере утончения пленки.
Следует подчеркнуть, что прочность покрытий CVD превосходила прочность типичных гидрофобных однослойных покрытий. Результаты [47] показали, что графен является многообещающим поверхностным покрытием для обеспечения капельной конденсации воды в промышленных условиях с возможностью масштабирования с помощью CVD. В последнее время для создания подобных покрытий стало возможным использовать и другие, более простые технологии [68–77].
В работе [45] покрытия из восстановленного оксида графена были изготовлены окунанием медных подложек в коллоидную суспензию оксида графена с последующим термическим восстановлением. На рис. 20 показано образование восстановленного оксида графена на поверхности (для доказательства этого факта были использованы методы комбинационного рассеяния света и рентгеновской фотоэлектронной спектроскопии). Применение сканирующей электронной и атомно-силовой микроскопии, а также исследование динамического контактного угла поверхности с водой позволили изучить морфологию и смачиваемость полученных покрытий.
Рис. 20.
Процесс нанесения графеновых покрытий для изучения капельной конденсации. а – формирование наноколлоидного графена; б – нанесение графена на подложки методом окунания; в – отжиг графенового слоя; г – общий вид капельной конденсации
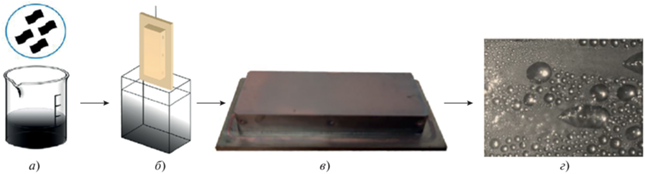
Такие покрытия использовались для исследования капельной конденсации чистого водяного пара и продемонстрировали уникальные результаты по сравнению с пленочной конденсацией. Кроме того, оказалось, что подобные покрытия весьма долговечны. На рис. 21 представлена зависимость коэффициента теплоотдачи при конденсации паров воды на поверхности из чистой меди, микрошероховатой меди и меди, покрытой графеновым слоем [45]. Нетрудно заметить, что при капельной конденсации на поверхности с графеновым покрытием коэффициент теплоотдачи в несколько раз превосходит таковой на поверхностях из чистой или микрошероховатой меди, тем более теплоотдачу при пленочной конденсации.
Рис. 21.
Зависимость коэффициента теплоотдачи от теплового потока при пленочной конденсации на поверхности меди (1) и капельной конденсации на поверхности меди, модифицированной графеном (2), чистой меди (3), микрошероховатой меди (4)
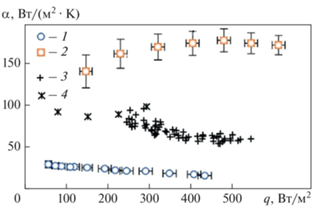
Как отметили авторы [45], графеновое покрытие оставалось стабильным более 100 ч при больших тепловых нагрузках. Этот результат находится в хорошем согласии с данными из [47], где капельной конденсации соответствовали устойчивые высокие показатели по теплоотдаче – более двух недель на покрытых графеном медных трубах в аналогичных условиях эксплуатации. Образцы также имели стабильные тепловые характеристики с практически постоянным коэффициентом теплоотдачи – около 165 кВт/(м2 ⋅ К).
ИСПОЛЬЗОВАНИЕ ГРАФЕНОВЫХ МАТЕРИАЛОВ В ТЕПЛОВЫХ ТРУБАХ И ТЕРМОСИФОНАХ
В этом разделе на примере конкретных технических устройств: тепловых труб и термосифонов – будут рассмотрены те преимущества, которые дает использование 2D-наноматериалов.
Как известно, тепловые трубы – одно из наиболее эффективных устройств для отвода тепла в различных системах. В настоящее время тепловые трубы обычно изготавливают из меди или алюминия. В работе [43] описан новый класс тепловых труб, имеющих существенно более высокие характеристики. На внутреннюю поверхность таких труб нанесена пленка графена с развитой наноструктурой (рис. 22).
Рис. 22.
Тепловая трубка с графеновым слоем [43]. 1 – графеновая пленка; 2 – углеродное волокно; 3 – пружина; 4 – рабочая жидкость
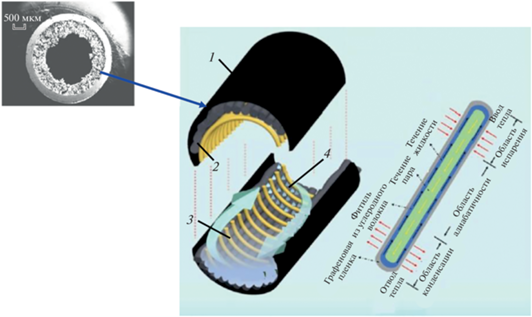
Графеновая тепловая труба состоит из трех ключевых компонентов – контейнера, фитиля особой конструкции и рабочей жидкости. Для повышения механической прочности контейнера в трубу вставлена медная пружина. С одной стороны, сверхвысокая теплопроводность графеновой пленки в плоскости обеспечивает быстрый перенос тепла от области испарителя в область конденсатора. С другой стороны, рабочая жидкость испаряется, когда тепло поступает в графеновую тепловую трубу, при этом испарившаяся жидкость создает градиент давления, чтобы заставить пар двигаться в область конденсации. Когда пар достигает области конденсации, производится отвод тепла. После этого рабочая жидкость пассивно перекачивается в испарительную область для повторного испарения посредством капиллярной силы фитиля наноструктуры. Следует отметить, что чешуйки графена должны быть уложены внутри пленки упорядоченно одна на другую для обеспечения высокой теплопроводности. Зависимость коэффициента теплопроводности пленки из упорядоченных слоев графена от ее толщины представлена на рис. 23 [78]. Хорошо видно, что при толщине пленки около 6 мкм продольная теплопроводность (вдоль слоев графена) составляет 2500 Вт/(м · К), а при толщине более 25 мкм – 1400 Вт/(м · К) (на графике не показано), что в несколько раз выше, чем у меди и алюминия.
Рис. 23.
Зависимость коэффициента теплопроводности графеновой пленки λ от толщины слоя δ. а, б – графеновая пленка после отжига при температуре 2850 и 1300°С; в, г – толстые и средней толщины графеновые пленки после отжига; 1, 2 – диффузионный и вакуумный отжиг графеновой пленки при температуре 2850°С; 3 – отжиг в воздухе при температуре 1300°С; 4 – чистая медь; 5 – чистый алюминий
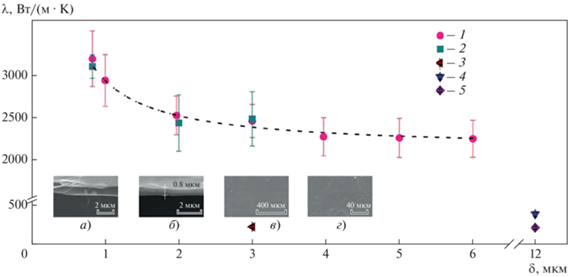
В результате в графеновой тепловой трубе коэффициент теплопередачи значительно увеличивается, достигая 7230 Вт/(м2 · K), что примерно в 3.5 раза выше, чем у коммерческих тепловых труб на основе меди. Такие рекордные показатели означают, что, используя двумерные графеновые структуры, можно существенно улучшить теплофизические параметры известных конструкций. Это открывает путь к созданию графеновых тепловых труб и внедрению их в легкие и мощные системы охлаждения, которые требуются во многих отраслях, например в энергетике, в автомобильной и космической электронике, при производстве телефонов и др.
В работе [44] на основе экспериментальных данных проанализирована теплопередача в миниатюрной петлевой тепловой трубе (МПТТ) с наножидкостью графен – вода (схема представлена на рис. 24). Использованная в исследовании труба состояла из квадратного плоского испарителя размером 20 × 20 мм, компенсационной камеры, расположенной над ним, и транспортных линий различного диаметра. Благодаря разнице в диаметрах исключалась возможность обратного потока пара через жидкостный трубопровод, а также увеличивалась скорость потока конденсата через него. Свойства графеновой наножидкости, отнесенные к свойствам базовой жидкости (воды), показаны на рис. 6.
Рис. 24.
Схема включения миниатюрной петлевой тепловой трубы. 1 – изолирующий блок; 2 – нагреватель; 3 – МПТТ; 4 – конденсатор; Т1–Т15 – термопары; V – вольтметр
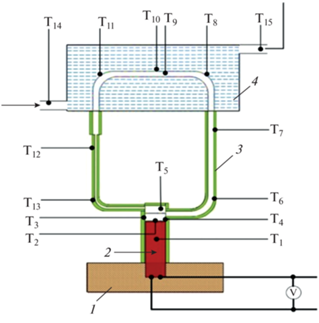
Во всех экспериментах использовалась оптимальная степень заполнения объема тепловой трубы – 30% общего показателя. Эксперименты проводились для диапазона тепловой нагрузки 20–380 Вт при вертикальной ориентации трубы. Нанолисты толщиной 1–5 нм с очень низкими объемными долями графена (0.003, 0.006 и 0.009%) смешивали с дистиллированной водой для приготовления наножидкости. Результаты опытов показали, что наножидкости улучшают тепловые характеристики миниатюрной петлевой тепловой трубы и снижают температуру поверхности испарителя по сравнению с дистиллированной водой. Коэффициент теплопередачи в МПТТ как функция тепловой нагрузки представлен на рис. 25.
Рис. 25.
Зависимость коэффициента теплоотдачи α в МПТТ от тепловой нагрузки Q. 1 – дистиллированная вода; вода + φ, % (по объему): 2 – 0.003; 3 – 0.006; 4 – 0.009
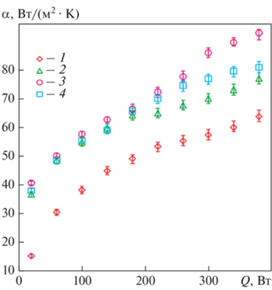
Найдена оптимальная концентрация графена – около 0.006%, которая обеспечивает максимальное улучшение теплопередачи. Наименьшее термическое сопротивление (0.083 К/Вт при 380 Вт) наблюдается при отмеченной оптимальной концентрации и на 21.6% меньше значения для дистиллированной воды. Температура поверхности испарителя достигла всего 106.3°C при 380 Вт, что на 10.3°C ниже, чем для дистиллированной воды. Результаты экспериментов подтверждают, что использование миниатюрной петлевой тепловой трубы, заполненной наножидкостью графен – вода, более эффективно для охлаждения, чем без этой наножидкости.
Недавно исследована интенсификация теплообмена в двухфазном закрытом термосифоне, покрытом графеновыми нанопластинками (GNF) различной смачиваемости [59]. Гидрофобные по своей сути графеновые нанопластинки можно модифицировать посредством простого процесса термического отверждения, чтобы они стали супергидрофильными.
Работа двухфазного закрытого термосифона определяется тремя основными процессами – испарением, конденсацией и циркуляцией конденсата (рис. 26). В [59] получены тепловые характеристики такого термосифона с покрытием из графеновых нанопластинок различной смачиваемости. Наделенные уникальным свойством быстрого проникновения воды, супергидрофильные графеновые нанокапилляры распространяют пленку воды на большую площадь поверхности, что способствует испарению. Максимальное улучшение эффективности достигается при использовании коэффициента теплоотдачи при испарении в качестве индикатора и его значение увеличивается при малых тепловых мощностях (менее 25 Вт) более чем в 15 раз, а при больших (свыше 30 Вт) – примерно в 2 раза (рис. 27). Показаны средние значения коэффициента теплопередачи испарителя (см. рис. 26, а; максимальный разброс данных составляет 11.96%) и конденсатора (см. рис. 26, б; максимальная погрешность равна 4.32%). Стоит отметить, что при конденсации рост коэффициента теплоотдачи имеет несколько иной характер (см. рис. 27, б).
Рис. 26.
Диаграммы работы двухфазного закрытого термосифона без покрытия (а), с покрытием из неотвержденного слоя GNF (вариант 1) (б) и из отвержденного слоя GNF (вариант 2) (в) [59], графеновое покрытие в конденсаторе (г) и испарителе (д). 1 – графеновое покрытие; 2, 3 – режим пленочного и пузырькового испарения; 4 – конденсация; 5 – испарение; 6 – ввод тепла; 7 – транспортирование воды
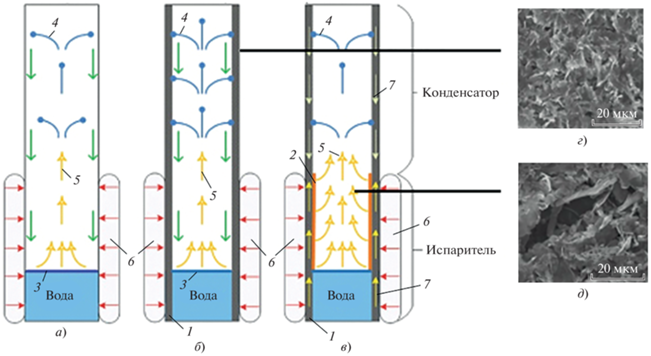
Рис. 27.
Зависимость коэффициента теплоотдачи при испарении (а) и конденсации (б) от тепловой нагрузки в двухфазном закрытом термосифоне без покрытия (1), с неотвержденным (2) и отвержденным (3) покрытием GNF
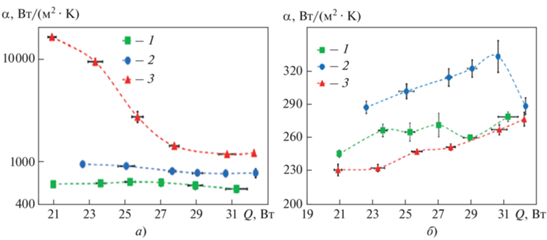
Неотвержденное гидрофобное покрытие хорошо подходит для процесса конденсации ввиду повышения теплоотдачи примерно на 20%. При оценке общего теплового сопротивления двухфазного закрытого термосифона можно добиться тепловой оптимизации путем изменения отношения длины испарителя к длине конденсатора.
Скорость переноса воды через графеновые нанокапилляры значительно увеличена по сравнению с этой скоростью в капиллярах микроскопического масштаба из-за преобладания исключительно высокого капиллярного давления и большой длины скольжения [9]. В работе [73] проведены исследования по интеграции графеновых нанокапилляров в микротепловую трубу для повышения эффективности охлаждения мощных светодиодов (light emitting diode – LED-охлаждение). Показано, что с помощью графеновых нанокапилляров можно добиться сверхбыстрого движения воды, усиленного синергетически улучшением циркуляции и испарением воды в микрофлюидном устройстве. Подобная микротруба с графеновым покрытием обеспечивает повышение общей производительности более чем на 45% по сравнению с ее аналогом без покрытия. В свою очередь, эксперименты демонстрируют резкое снижение рабочей температуры светодиода (более чем на 25°C), что приводит к существенному увеличению срока его службы.
Подробное объяснение этого эффекта дано в [74], где описан эффект нетривиальной капиллярной термогидродинамики в ламинарных нанопористых графеновых структурах для систем термоменеджмента и теоретически предсказаны давления до 300 МПа и скорость потока воды более 35 м/с, что в 80 раз превышает скорость воды, если вычислять ее по соотношению Пуазейля для течения. При проведении молекулярно-динамического моделирования в работе [74] было установлено, что оксигенированные функциональные группы, прикрепленные к графену, дополнительно повышают капиллярное давление (около 100 МПа) и эффективную скорость (примерно 20 м/с) воды по сравнению с таковыми (примерно 50 МПа и 10 м/с) в чистом графеновом наноканале. Указанные эффекты дают возможность лучше понять феномен сверхбыстрого переноса воды в графеновых нанокапиллярах. Такая их способность позволяет в десятки раз увеличить скорость испарения с наноразмерных менисков, что подтверждается результатами и других исследований [6, 9, 37] и лишний раз свидетельствует об огромном потенциале приложений наноматериалов для управления потоками тепловой энергии, охлаждения энергетических и электронных устройств, изготовления солнечных панелей и батарей для преобразования солнечной энергии [9, 75–82], а также в системах очистки и обессоливания воды.
Необходимо дальнейшее изучение описанных в статье впечатляющих возможностей использования двумерных материалов в энергетических технологиях, особенно стабильности таких материалов и воспроизводимости получаемых результатов. В некоторых случаях требуется применение определенных методов стабилизации. В частности, для наножидкостей используются поверхностно-активные вещества, для обработки поверхностей с покрытиями из 2D-материалов – отжиг или аналогичные технологии. Следует обратить внимание на то, что при нагреве до достаточно высоких температур (вплоть до 700°С) твердые графеновые покрытия и пленки стабильны в течение десятков часов без потери основных тепломассообменных характеристик. В некоторых цитируемых в статье работах [10, 17, 18, 36, 37, 41, 42, 44, 45] эти вопросы рассмотрены довольно подробно. Проблемы стабильности и воспроизводимости материалов и изделий из них обсуждаются также в обзорах [83–86].
ВЫВОДЫ
1. Двумерные наноматериалы обладают уникальными свойствами, открывающими интересные перспективы для их применения в энергетическом оборудовании при переносе тепла. Семейство таких материалов постоянно расширяется вследствие не только вариаций на основе графена, но и создания принципиально новых материалов, построенных из других химических элементов и соединений.
2. Существуют два основных способа применения 2D-материалов для интенсификации процессов теплопередачи – получение и использование наножидкостей в качестве теплоносителей и нанесение различного рода покрытий из 2D-материалов на поверхности теплообмена.
3. Использование двумерных наноматериалов позволяет заметно интенсифицировать процессы переноса тепла при однофазной конвекции, испарении, кипении, конденсации. Экспериментально подтверждается, что эти материалы могут с успехом применяться в реальных конструкциях теплообменных устройств (тепловых трубах, термосифонах).
Список литературы
Advanced materials for our energy future: Booklet / Materials Research Society, 2010.
Advanced materials for clean and sustainable energy and mobility / M. Ierides, R. de Valle, D. Fernandez, L. Bax, P. Jacques, F. Stassin, M. Meeus. EMIRI, 2019.
Total material requirement for the global energy transition to 2050: A focus on transport and electricity / T. Watari, B.C. McLellan, D. Giurco, E. Dominish, E. Yamasue, K. Nansai // Resour., Conserv. Recycl. 2019. V. 148. P. 91–103. https://doi.org/10.1016/j.resconrec.2019.05.015
Materials challenges for nuclear systems / T. Allen, J. Busby, M. Meyer, D. Petti // Mater. Today. 2010. V. 13. Is. 12. P. 14–23. https://doi.org/10.1016/S1369-7021(10)70220-0
Дмитриев А.С., Клименко А.В. Преобразование солнечного излучения в пар – новые возможности на основе наноматериалов (обзор) // Теплоэнергетика. 2020. № 2. С. 3–19. https://doi.org/10.1134/S0040363620020010
Дмитриев А.С., Михайлова И.А. Введение в наноэнергетику: учеб. пособие. М.: Издательский дом МЭИ, 2011.
Дмитриев А.С., Михайлова И.А. Физико-химия наноструктур. М.: Издательский дом МЭИ, 2013.
Дмитриев А.С. Теплофизические проблемы наноэнергетики. Часть 1, 2 // Теплоэнергетика. 2010. № 12. С. 13 –22; 2011. № 4. С. 29–36.
Дмитриев А.С. Введение в нанотеплофизику. М.: БИНОМ, 2015.
Emerging beyond-graphene elemental 2D materials for energy and catalysis applications / F. Fan, R. Wang, H. Zhang, W. Wu // Chem. Soc. Rev. 2021. V. 50. Is. 19. P. 10983–11031. https://doi.org/10.1039/C9CS00821G
Vishnoi P., Pramoda K., Rao C.N.R. 2D elemental nanomaterials beyond graphene // ChemNanoMat. 2019. V. 5. Is. 9. P. 1062–1091. https://doi.org/10.1002/cnma.201900176
Two-dimensional nanocrystals produced by exfoliation of Ti3AlC2 / M. Naguib, M. Kurtoglu, V. Presser, J. Lu, J. Niu, M. Heon, L. Hultman, Y. Gogotsi, M. Barsoum // Adv. Mater. 2011. V. 23. Is. 37. P. 4248–4253. https://doi.org/10.1002/adma.201102306
Najafabadi A.T. Emerging applications of graphene and its derivatives in carbon capture and conversion: current status and future prospects // Renewable Sustainable Energy Rev. 2015. V. 41. P. 1515–1545. https://doi.org/10.1016/j.rser.2014.09.022
Structure-dependent electrical properties of graphene nanoribbon devices with graphene electrodes / L. Martini, Z. Chen, N. Mishra, N. Mishra, G.B. Barin, P. Fantuzzi, P. Ruffieux, R. Fasel, X. Feng, A. Narita, C. Coletti, K. Müllen, A. Candini // Carbon. 2019. V. 146. P. 36–43. https://doi.org/10.1016/j.carbon.2019.01.071
Iwan A., Malinowski M., Pasciak G. Polymer fuel cell components modified by graphene: electrodes, electrolytes and bipolar plates // Renewable Sustainable Energy Rev. 2015. V. 49. P. 954–967. https://doi.org/10.1016/j.rser.2015.04.093
2D materials as an emerging platform for nanopore- based power generation / M. Macha, S. Marion, V.V.R. Nandigana, A. Radenovic // Nat. Rev. Mater. 2019. V. 4. P. 588–605. https://doi.org/10.1038/s41578-019-0126-z
Graphene, related two-dimensional crystals, and hybrid systems for energy conversion and storage / F. Bonaccorso, L. Colombo, G. Yu, M. Stoller, V. Tozzini, A.C. Ferrari, R.S. Ruoff, V. Pellegrini // Sci. 2015. V. 347. Is. 6217. P. 1246501. https://doi.org/10.1126/science.1246501
Graphene and graphene-like materials for hydrogen energy / O.K. Alekseeva, I.V. Pushkareva, A.S. Pushkarev, V.N. Fateev // Nanotechnol. in Russia. 2020. V. 15. Is. 3. P. 273–300. https://doi.org/10.1134/S1995078020030027
Deng S., Berry V. Wrinkled, rippled and crumpled graphene: an overview of formation mechanism, electronic properties, and applications // Mater. Today. 2016. V. 19. Is. P. 197–212. https://doi.org/10.1016/j.mattod.2015.10.002
Larcher D., Tarascon J.-M. Towards greener and more sustainable batteries for electrical energy storage // Nat. Chem. 2015. V. 7. P. 19–29. https://doi.org/10.1038/nchem.2085
Lee S., Cho J. Critical requirements for rapid charging of rechargeable Al- and Li-ion batteries // Angew. Chem. 2015. V. 54. Is. 33. P. 9452–9455. https://doi.org/10.1002/anie.201504466
Whittingham M.S. Lithium batteries and cathode materials // Chem. Rev. 2004. V. 104. Is. 10. P. 4271–4302. https://doi.org/10.1021/cr020731c
Visualization and quantification of electrochemical and mechanical degradation in Li-ion batteries / M. Ebner, F. Marone, M. Stampanoni, V. Wood // Sci. 2013. V. 342. Is. 6159. P. 716–720. https://doi.org/10.1126/science.1241882
The chemistry of two-dimensional layered transition metal dichalcogenide nanosheets / M. Chhowalla, H. Shin, G. Eda, L.-J. Li, K.P. Loh, H. Zhang // Nat. Chem. 2013. V. 5. P. 263–275. https://doi.org/10.1038/nchem.1589
Pumera M., Sofer Z., Ambrosi A. Layered transition metal dichalcogenides for electrochemical energy generation and storage // J. Mater. Chem. A. 2014. V. 2. Is. 24. P. 8981–8987. https://doi.org/10.1039/C4TA00652F
25th anniversary article: MXenes: a new family of two-dimensional materials / M. Naguib, V. Mochalin, M. Barsoum, Y. Gogotsi // Adv. Mater. 2014. V. 26. Is. 7. 992–1005. https://doi.org/10.1002/adma.201304138
Layered lithium transition metal oxide cathodes towards high energy lithium-ion batteries / P. He, H. Yu, D. Li, H. Zhou // J. Mater. Chem. 2012. V. 22. Is. 9. P. 3680–3695. https://doi.org/10.1039/C2JM14305D
Layered oxides as positive electrode materials for N-a‑ion batteries / K. Kubota, N. Yabuuchi, H. Yoshida, M. Dahbi, S. Komaba // MRS Bull. 2014. V. 39. P. 416–422. https://doi.org/10.1557/mrs.2014.85
Enabling sodium batteries using lithium-substituted sodium layered transition metal oxide cathodes / D. Kim, S.-H. Kang, M. Slater, S. Rood, J.T. Vaughey, N. Karan, M. Balasubramanian, C.S. Johnson // Adv. Energy Mater. 2011. V. 1. Is. 3. P. 333–336. https://doi.org/10.1002/aenm.201000061
Hynek D., Pondick J., Cha J. The development of 2D materials for electrochemical energy applications: A mechanistic approach // APL Mater. 2019. V. 7. Is. 3. P. 030902. https://doi.org/10.1063/1.5085187
Ni W., Shi L. Layer-structured carbonaceous materials for advanced Li-ion and Na-ion batteries: Beyond graphene: a review article // J. Vacuum Sci. Technol. A. 2019. V. 37. Is. 4. P. 040803. https://doi.org/10.1116/1.5095413
Two-dimensional material-based heterostructures for rechargeable batteries / Z. Hu, Q. Liu, S.-L. Chou, S.-X. Dou // Cell Rep. Phys. Sci. 2021. V. 2. Is. 1. P. 100286. https://doi.org/10.1016/j.xcrp.2020.100286
Lu Y., Yu L., Lou X. Nanostructured conversion-type anode materials for advanced lithium-ion batteries // Chem. 2018. V. 4. Is. 5. P. 972–996. https://doi.org/10.1016/j.chempr.2018.01.003
Two-dimensional material separation membranes for renewable energy purification, storage, and conversion / L. Dai, K. Huang, Y. Xia, Z. Xu // Green Energy Environ. 2021. V. 6. Is. 2. P. 193–211. https://doi.org/10.1016/j.gee.2020.09.015
Materials for future nuclear energy systems / G.S. Was, D. Petti, S. Ukai, S. Zinkle // J. Nucl. Mater. 2019. V. 527. P. 151837.https://doi.org/10.1016/j.jnucmat.2019.151837
Torii S. Enhancement of heat transfer performance in pipe flow using graphene-oxide-nanofluid and its application // Mater. Today: Proc. 2021. V. 35. Part 3. P. 506–511. https://doi.org/10.1016/j.matpr.2020.04.078
Dmitriev A.S. Hybrid graphene nanocomposites: thermal interface materials and functional energy materials in: graphene production and application // Graphene Production and Application / Ed. by S. Ameen, M.S. Akhtar, H.-S. Shin. IntechOpen, 2019. https://doi.org/10.5772/intechopen.89631
Role of graphene nanofluids on heat transfer enhancement in thermosiphon / S. Das, A. Giri, S. Samanta, S. Kanagaraj // J. Sci.: Adv. Mater. Devices. 2019. V. 4. Is.1. P. 163–169. https://doi.org/10.1016/j.jsamd.2019.01.005
Graphene-water nanofluid in heat exchanger: Mathematical modelling, simulation and economic evaluation / D. Purbia, A. Khandelwal, A. Kumar, A. Sharma // Int. Commun. Heat Mass Transfer. 2019. V. 108. P. 104327. https://doi.org/10.1016/j.icheatmasstransfer.2019.104327
Keklikcioglu O., Dagdevir T., Ozceyhan V. Heat transfer and pressure drop investigation of graphene nanoplatelet-water and titanium dioxide-water nanofluids in a horizontal tube // Appl. Therm. Eng. 2019. V. 162. Is. 5. P. 114256. https://doi.org/10.1016/j.applthermaleng.2019.114256
Vishnuprasad S., Haribabu K., Perarasu V.T. Experimental study on the convective heat transfer performance and pressure drop of functionalized graphene nanofluids in electronics cooling system // Heat Mass Transfer. 2019. V. 55. Is. 8. P. 2221–2234. https://doi.org/10.1007/s00231-019-02581-6
An optimized graphene oxide self-assembly surface for significantly enhanced boiling heat transfer / W. Zhou, L. Mao, X. Hu, Y. He // Carbon. 2019. V. 50. P. 168–178. https://doi.org/10.1016/j.carbon.2019.04.119
A lightweight and high thermal performance graphene heat pipe / Y. Liu, S. Chen, Y. Fu, N. Wang, D. Mencarelli, L. Pierantoni, H. Lu, J. Liu // Nano Sel. 2020. V. 2. Is. 2. P. 364–372. https://doi.org/10.1002/nano.202000195
Thermal performance of miniature loop heat pipe with graphene–water nanofluid / T. Tharayil, L. Asirvatham, V. Ravindran, S. Wongwises // Int. J. Heat Mass Transfer. 2016. V. 93. P. 957–968. https://doi.org/10.1016/j.ijheatmasstransfer.2015.11.011
Solution-processed graphene oxide coatings for enhanced heat transfer during dropwise condensation of steam / E. Colusso, M. Tancon, L. Cazzola, R. Parin, S. Agnoli, F. De Boni, M.G. Pelizzo, E.D. Gaspera, D. Del Col, A. Martucci // Nano Sel. 2021. V. 2. Is. 1. P. 61–71. https://doi.org/10.1002/nano.202000105
Investigation of structure-property-boiling enhancement mechanisms of copper/graphene nanoplatelets coatings / A.M. Rishi, S.A. Rozati, C. Trybus, S.G. Kandlikar, A. Gupta // Front. Mech. Eng. 2021. V. 2. P. 642214. https://doi.org/10.3389/fmech.2021.642214
Scalable graphene coatings for enhanced condensation heat transfer / D.J. Preston, D.L. Mafra, N. Miljkovic, J. Kong, E.N. Wang // Nano Lett. 2015. V. 15. Is. 5. P. 2902–2909. https://doi.org/10.1021/nl504628s
Jaikumar A., Kandlikar S., Gupta A. Pool boiling enhancement through graphene and graphene oxide coatings // Heat Transfer Eng. 2017. V. 38. Is. 14–15. [Selected papers from the 13th Intern. Conf. on Nanochannels, Microchannels and Minichanles. San Francisco, California, USA, 6–9 July 2015.] P. 1274–1284. https://doi.org/10.1080/01457632.2016.1242959
Lee M., Heo H., Bang I. Effect of thermal activity on critical heat flux enhancement in downward-hemispherical surface using graphene oxide coating // Int. J. Heat Mass Transfer. 2018. V. 127. Part B. P. 1102–1111. https://doi.org/10.1016/j.ijheatmasstransfer.2018.07.151
Kim T., Park H., Chang S. CHF experiments using a 2-D curved test section with additives for IVR-ERVC strategy // Nucl. Eng. Des. 2021. V. 243. P. 272–278. https://doi.org/10.1016/j.nucengdes.2011.11.031
Comparison between nucleate pool boiling heat transfer of graphene nanoplatelet- and carbon nanotube-based aqueous nanofluids / A. Akbari, E. Mohammadian, S.A.A. Fazel, M. Shanbedi, M. Bahreini, M. Heidari, G. Ahmadi // ACS Omega. 2019. V. 4. Is. 21. P. 19183–19192. https://doi.org/10.1021/acsomega.9b02474
Gnielinski V. New equations for heat and mass transfer in turbulent pipe and channel flow // Int. Chem. Eng. 1979. V. 16. Is. 2. P. 359–368.
Experimental investigation on thermophysical properties of Therminol® 55 based hybrid nanofluids with alumina and graphene nanoplatelets for medium temperature applications / S. Shajan, V. Baiju, T.S. Krishnakumar, A. Godwin, T. Linia, A. Manu, M.B. Safeer // Therm. Sci. Eng. Prog. 2021. V. 26. P. 101116. https://doi.org/10.1016/j.tsep.2021.101116
Graphene-water nanofluid in heat exchanger: Mathematical modelling, simulation and economic evaluation / D. Purbia, A. Khandelwal, A. Kumar, A. Sharma // Int. Commun. Heat Mass Transfer. 2019. V. 108. P. 104327. https://doi.org/10.1016/j.icheatmasstransfer.2019.104327
Effect of thermal conductivity on enhanced evaporation of water droplets from heated graphene – PDMS composite surfaces / P. Goel, M. Choudhury, A. Aqeel, X. Li, L.-H. Shao, H. Duan // Langmuir. 2019. V. 35. Is. 21. P. 6916–6921. https://doi.org/10.1021/acs.langmuir.9b00799
Heat transfer enhancement of a multilayer graphene coating surface / C.-H. Wu, Y.A. Lee, Y.J. Lai, J. Shieh, C.R. Yang, C.-C. Hsu // Exp. Therm. Fluid Sci. 2020. V. 118. P. 110175. https://doi.org/10.1016/j.expthermflusci.2020.110175
Nucleate pool boiling enhancement by ultrafast water permeation in graphene-nanostructure / K.K. Lay, J.S. Ong, K.Y. Yong, M.K. Tan, Y.M. Hung // Int. Commun. Heat Mass Transfer. 2019. V. 101. P. 26–34. https://doi.org/10.1016/j.icheatmasstransfer.2018.12.015
Sezer N., Khan S., Koç M. Boiling heat transfer enhancement by self-assembled graphene/silver hybrid film for the thermal management of concentrated photovoltaics // Energy Technol. 2020. V. 8. Is. 11. P. 2000532. https://doi.org/10.1002/ente.202000532
Thermal performance enhancement and optimization of two-phase closed thermosyphon with graphene-nanoplatelets coatings / V.O. Ng, H. Yu, H.A. Wu, Y.M. Hung // Energy Convers. Manage. 2021. V. 236. P. 114039. https://doi.org/10.1016/j.enconman.2021.114039
Thermohydraulic analysis of covalent and noncovalent functionalized graphene nanoplatelets in circular tube fitted with turbulators / H. Tao, O.A. Alawi, O.A. Hussein, W. Ahmed, A.H. Abdelrazek, R.Z. Homod, M. Eltaweel, M.W. Falah, N. Al-Ansari, Z.M. Yaseen // Sci. Rep. 2022. V. 12. P. 17710. https://doi.org/10.1038/s41598-022-22315-9
Thermal performance of hybrid thermal interface graphene nanocomposites / D.D. Babenko, A.A. Dmitriev, A.S. Dmitriev, I.A. Mikhailova // J. Phys.: Conf. Ser. 2020. V. 1683. Is. 3. P. 032041 https://doi.org/10.1088/1742-6596/1683/3/032041
Babenko D.D., Dmitriev A.S., Mikhailova I.A. Thermohydrodynamic processes of droplet evaporation and boiling on functional nanomaterials surfaces // J. Phys.: Conf. Ser. 2020. V. 1565. [All-Russian scientific conf. with international participation “Thermophysics and Power Engineering in Academic Centers” (TPEAC-2019). St. Petersburg, Russia, 21–23 Oct. 2019.] P. 012010. https://doi.org/10.1088/1742-6596/1565/1/012010
Physical features of Leidenfrost effect on the surface of a graphene nanocomposite for the problems of thermal and nuclear energy / D.D. Babenko, A.S. Dmitriev, V.V. Mikhailov, I.A. Mikhailova // IOP Conf. Series: Mater. Sci. Eng. 2018. V. 447. P. 012044. https://doi.org/10.1088/1757-899X/447/1/012044
Dmitriev A.A., Dmitriev A.S., Mikhailova I. New nanocomposite thermal interface materials based on graphene flakes, mesoscopic microspheres and polymers // MATEC Web Conf. 2018. V. 207. Is. 2. P. 04002. https://doi.org/10.1051/matecconf/201820704002
Dmitriev A.S., Valeev A.R. Graphene nanocomposites as thermal interface materials for cooling energy devices // J. Phys.: Conf. Ser. 2017. V. 891. [The Intern. Conf. “Problems of Thermal Physics and Power Engineering” (PTPPE-2017). Moscow, Russia, 9–11 Oct. 2017.] P. 012359. https://doi.org/10.1088/1742-6596/891/1/012359
Dmitriev A.S. Non-trivial capillary hydrodynamics and strong evaporative cooling in multilayer two-dimensional materials: application in thermal management systems of electronic and energy devices // J. Phys.: Conf. Ser. 2021. V. 2039. [XXIII School-Seminar of young scientists and specialists under the leadership of the academician, professor A.I. Leontiev “Problems of Heat and Mass Transfer and Gas Dynamics in Power Plants” (XXIII SSYSS 2021). Yekaterinburg, Russia, 24–28 May 2021.] P. 012008. https://doi.org/10.1088/1742-6596/2039/1/012008.A.S
Goh J.Y.H., Hung Y.M., Tan M.K. Extraordinarily enhanced evaporation of water droplets on graphene-nanostructured coated surfaces // Int. J. Heat Mass Transfer. 2020. V. 163. P. 120396. https://doi.org/10.1016/j.ijheatmasstransfer.2020.120396
Graphene-based devices for thermal energy conversion and utilization / Y.-T. Li, Y. Tian, M.-X. Sun, T. Tu, Z.-Y. Ju, G.-Y. Gou, Y.-F. Zhao, Z.-Y. Yan, F. Wu, D. Xie, H. Tian, Y. Yang, T.-L. Ren // Adv. Funct. Mater. 2019. V. 30. Is. 8. P. 1903888. https://doi.org/10.1002/adfm.201903888
Recent developments, challenges, and pathways to stable dropwise condensation: A perspective / J. Ma, S. Sett, H. Cha, X. Yan, N. Miljkovic // Appl. Phys. Lett. 2020. V. 116. Is. 8. P. 260501. https://doi.org/10.1063/5.0011642
Sustaining dropwise condensation on nickel-plated copper surfaces with as-grown graphene coatings / W. Chang, K. Luo, P. Wang, C. Li // Appl. Therm. Eng. 2022. V. 209. P. 118319. https://doi.org/10.1016/j.applthermaleng.2022.118319
Few-layer graphene on nickel enabled sustainable dropwise condensation / W. Chang, B. Peng, K. Egab, Y. Zhang, Y. Cheng, X. Li, X. Ma, C. Li // Sci. Bull. 2021. V. 66. Is. 18. P. 1877–1884. https://doi.org/10.1016/j.scib.2021.06.006
Haque M.R., Das S.R., Betz A.R. Experimental investigation of condensation and freezing phenomena on hydrophilic and hydrophobic graphene coating // Appl. Therm. Eng. 2019. V. 160. P. 113987. https://doi.org/10.1016/j.applthermaleng.2019.113987
Grain size effects on the wettability of as-grown graphene and dropwise condensation / W. Chang, B. Peng, A.S. Khan, M. Alwazzan, Y. Zhang, X. Li, Y. Tong, C. Li // Carbon. 2021. V. 171. P. 507–513. https://doi.org/10.1016/j.carbon.2020.09.025
Enhanced heat transport behavior of micro channel heat sink with graphene based nanofluids / T. Balaji, C. Selvam, D.M. Lal, S. Harish // Int. Commun. Heat Mass Transfer. 2020. V. 117. P. 104716. https://doi.org/10.1016/j.icheatmasstransfer.2020.104716
A review on graphene based nanofluids: Preparation, characterization and applications / A. Arshad, M. Jabbal, Y. Yan, D. Reay // J. Mol. Liq. 2019. V. 279. P. 444–484. https://doi.org/10.1016/j.molliq.2019.01.153
Thermophysical performance of graphene based aqueous nanofluids / Y. Wang, H.A.I. Al-Saaidi, M. Kong, J.L. Alvarado // Int. J. Heat Mass Transfer. 2018. V. 119. P. 408–417. https://doi.org/10.1016/j.ijheatmasstransfer.2017.11.019
Review on the recent progress in the preparation and stability of graphene‑based nanofluids / T.L. Ba, O. Mahian, S. Wongwises, I.M. Szilagyi // J. Therm. Anal. Calorimetry. 2020. V. 142. P. 1145–1172. https://doi.org/10.1007/s10973-020-09365-9
Enhanced boiling heat transfer by nucleation patterning with self-assembly of reduced graphene oxide coating / G. Choi, M. Yun, W.-T. Hsu, D. Shim, D. Lee, B.S. Kim, H.H. Cho // Int. J. Heat Mass Transfer. 2022. V. 197. P. 123329. https://doi.org/10.1016/j.ijheatmasstransfer.2022.123329
Performance enhancement of graphene-coated micro heat pipes for light-emitting diode cooling / J.S. Gan, H. Yu, M.K. Tan, A.K. Soh, H.A. Wu, Y.M. Hung // Int. J. Heat Mass Transfer. 2020. V. 154. P. 119687. https://doi.org/10.1016/j.ijheatmasstransfer.2020.119687
Dmitriev A.S., Klimenko A.V. On thermal problems of the solar thermal multigeneration: new nanomaterials and working fluids // J. Phys.: Conf. Ser. 2022. V. 2150. [XII All Russian conf. “Thermophysics and Power Engineering in Academic Centers” (TPEAC 2021). Sochi, Russia, 25–27 Oct. 2021.] P. 012006. https://doi.org/10.1088/1742-6596/2150/1/012006
On the dynamic effects of evaporation and boiling of drops on inhomogeneous graphene substrates for effective thermal management of energy devices / D.D. Babenko, A.S. Dmitriev, V.V. Mikhailov, I.A. Mikhailova // J. Phys.: Conf. Ser. 2021. V. 2039. [XXIII School-seminar of young scientists and specialists under the leadership of the academician, professor A.I. Leontiev “Problems of Heat and Mass Transfer and Gas Dynamics in Power Plants” (XXIII SSYSS 2021). Yekaterinburg, Russia, 24–28 May 2021.] P. 012002. https://doi.org/10.1088/1742-6596/2039/1/012002
Structured graphene metamaterial selective absorbers for high efficiency and omnidirectional solar thermal energy conversion / K.-T. Lin, H. Lin, T. Yang, B. Jia // Nat. Commun. 2020. V. 11. P. 1389. https://doi.org//10.1038/s41467-020-15116-z
Recent progress in two dimensional Mxenes for photocatalysis: A critical review / T. Haneef, K. Rasoo, J. Iqba, R. Nawaz, M.R.U. Mustafa, K.A. Mahmoud, T. Sarkar, A. Shahzad // 2D Mater. 2022. V. 10. Is. 1. P. 012001. https://doi.org/10.1088/2053-1583/ac9e66
2D molybdenum and vanadium nitrides synthesized by ammoniation of 2D transition metal carbides (MXenes) / P. Urbankowski, B. Anasori, K. Hantanasirisakul, L. Yang, L. Zhang, B. Haines, S.J. May, S.J.L. Billinge, Y. Gogotsi // Nanoscale. 2017. V. 9. Is. 45. P. 17722–17730. https://doi.org/10.1039/C7NR06721F
Application of graphene in energy storage device – A review / A. Olabi, M. Abdelkareem, T. Wilberforce, E. Sayed // Renewable Sustainable Energy Rev. 2021. V. 135. P. 110026. https://doi.org/10.1016/j.rser.2020.110026
An advanced electrocatalyst with exceptional eletrocatalytic activity via ultrafine Pt-based trimetallic nanoparticles on pristine graphene / J. Zhao, H. Li, Z. Liu, W. Hu, C. Zhao, D. Shi // Carbon. 2015. V. 87. P. 116–127. https://doi.org/10.1016/j.carbon.2015.01.038
Дополнительные материалы отсутствуют.
Инструменты
Теплоэнергетика