Журнал высшей нервной деятельности им. И.П. Павлова, 2023, T. 73, № 4, стр. 524-536
Обратные тормозные связи через ГАМК-Б-рецепторы синхронизируют интериктальные разряды в коре
В. Г. Марченко 1, *, М. И. Зайченко 1, **
1 Федеральное государственное бюджетное учреждение науки
Институт высшей нервной деятельности и нейрофизиологии РАН
Москва, Россия
* E-mail: vgmarchenko3@gmail.com
** E-mail: mariya-zajchenko@yandex.ru
Поступила в редакцию 15.09.2022
После доработки 23.01.2023
Принята к публикации 27.02.2023
- EDN: WHMBJJ
- DOI: 10.31857/S004446772304007X
Аннотация
Современные экспериментальные данные показывают, что интериктальные разряды состоят из короткого спайка и медленной волны, которая рассматривается как длительная гиперполяризация. На модели синхронизации интериктальных разрядов было проведено исследование обратных тормозных связей. У крыс, находящихся в состоянии легкого наркотического сна, после нанесения блокаторов ГАМК-А-рецепторов на кору интериктальные разряды возникали в соседних участках коры независимо друг от друга, а затем происходила их синхронизация. В экспериментах, в которых интериктальные разряды возникали одновременно, длительности медленной волны (тормозной фазы) были одинаковы. В процессе регистрации в этих и других опытах происходило увеличение временно́й задержки между моментами генерации интериктальных разрядов. В условиях усиления синхронизации происходило увеличение длительности медленной волны (тормозной фазы) интериктальных разрядов. У интериктальных разрядов, которые возникали первыми, наблюдалась больша́я длительность торможения по сравнению с длительностью в соседней точке, так как к собственному торможению в нейронной сети добавляется торможение от соседних сетей по обратным тормозным связям. При возникновении возбуждения вслед за ним по обратной связи начиналось торможение, которое ограничивало период возбуждения, и таким образом создавалось временно́е окно интеграции, и это также происходило в соседних нейронных сетях коры.
Пространственно-временна́я синхронизированная активность нейронов играет центральную роль в ощущениях, движении и познании (Knoblich et al., 2019; Trevelyan, 2016). Процессы интеграции и взаимодействия происходят при синхронизации электрической активности нейронных сетей коры, которые расположены в одной или нескольких взаимосвязанных структурах мозга (Lévesque et al., 2019). Синхронная активность нейронных сетей в физиологических условиях отражается специфическими осцилляторными паттернами ЭЭГ, которые ассоциируются с различными поведенческими состояниями и когнитивными функциями, однако чрезмерная синхронизация может привести к фокальной эпилептиформной активности, характеризующейся интериктальными и иктальными разрядами у больных эпилепсией и в моделях на животных (Lévesque et al., 2019).
В коре существует несколько механизмов взаимодействия между нейронными сетями, которое находит свое отражение в синхронизации электрической активности, – через электрические синапсы, посредством электрических полей, объемной передачи, через общий вход, посредством обратной связи между нейронами и созданием временного окна интеграции (Gray, Singer, 1989). Причем это взаимодействие осуществляется как в локальном пуле клеток коры, так и между удаленными областями. Ключевую роль в этом взаимодействии играет процесс торможения, напрямую осуществляя синхронизацию нейронов, определяя параметры колебаний и поддерживая их когерентность в пространстве (Isaacson, Scanziani, 2011). Синхронное ГАМК-ергическое торможение является мощным механизмом синхронизации нейронов при генерации физиологических паттернов активности. Механизм синхронизации посредством торможения действительно работает во время генерации некоторых типов эпилептиформной активности (Khazipov, 2016).
Основным тормозным нейромедиатором в мозге является ГАМК. Интернейроны могут участвовать в синхронизации нейронных сетей, действуя не только через ГАМК-А-, но и через ГАМК-Б-рецепторы. Активация постсинаптической калиевой проводимости ГАМК-Б-рецепторами включает G-белок-опосредованные каскады, и поэтому тормозные постсинаптические потенциалы (ТПСП), опосредованные ГАМК-Б-рецепторами, медленные, длятся сотни миллисекунд (Khazipov, 2016).
Для нас наибольший интерес представляло торможение посредством ГАМК-Б-рецепторов, поэтому исследование механизмов синхронизации интериктальных спайков проводилось нами в ситуации, когда ГАМК-А-рецепторы были заблокированы. При блокировании ГАМК-А-рецепторов в коре возникают интериктальные спайки, которые в дальнейшем синхронизируются. Такая экспериментальная модель является очень удобной для исследования механизмов синхронизации (Марченко, Зайченко, 2015).
Было обнаружено, что активация ГАМК-Б-рецепторов в периформной коре имеет двойные эффекты in vivo – с одной стороны, подавляет спонтанную активность в пирамидных клетках, а с другой стороны, способствует периодической синхронной эпилептиформной активности в тех же клетках (Gerrard et al., 2018; Lévesque et al., 2019; Jurado-Parras et al., 2016).
ГАМК-Б-рецепторы принимали непосредственное участие в генерации медленной активности (Serrats et al., 2017). Медленная кинетика рецепторов ГАМК-Б аналогична медленным течениям состояний Up и Down, позволяя рецепторам ГАМК-Б оказывать сильный синаптический эффект и таким образом контролировать частоту и синхронизацию медленных колебаний (Wang, 2010).
Еще одним важным механизмом для синхронизации является электрический контакт через щелевые соединения каналов между нейронами (gap junctions) (Wang, Wu, 2021). Следует отметить, что такой механизм синхронизации работает даже при блокаде ГАМК-А-рецепторов (Gibson et al., 2005). Электрические синапсы могут опосредовать сильную и точную во времени (обычно до ±1 мс, но возможно <1 мкс в благоприятных нейронных системах) синхронизацию как подпороговых мембранных потенциалов, так и потенциалов действия при соответствующих условиях (Connors, 2017).
Велика роль электрических синапсов в эпилептогенезе, так как они образуют прямые межклеточные связи между нейронами, способствуя гиперсинхронности нейронной активности, связанной с судорогами (Li et al., 2019). Синхронизация активности в корковых нейронных сетях осуществляется посредством электрических синапсов интернейронов. Электрические синапсы могут играть важную роль в синхронизации нейронных сетей в физиологических и патологических условиях, включая эпилептические расстройства (Lévesque et al., 2019).
Синхронизация может осуществляться и посредством полевых потенциалов (эфаптическая связь). Этот полевой потенциал имеет большую амплитуду и распространяется на большие расстояния по коре практически мгновенно (Марченко и др., 2018).
Наряду с другими показателями эпилептической активности, важным компонентом являются интериктальные разряды (Ding et al., 2019). Интериктальные спайки наблюдаются и при экспериментальной эпилепсии у животных. Они появляются при нанесении блокаторов ГАМК-А-рецепторов на кору мозга – пенициллина (Марченко и др., 2018), бикукуллина (Zheng et al., 2020), пикротоксина (Bink et al., 2018), которые блокируют синаптическое торможение, а также 4-аминопиридина (4-АР) (Köhling et al., 2016; Amakhin et al., 2016). Запускать интериктальные спайки может также специфическая фотостимуляция парвальбуминовых (PV) или соматостатиновых (SOM) интернейронов (Yekhlef et al., 2015). Интериктальные разряды, регистрируемые в кортикальных нейронах, проявляются пароксизмальными деполяризующими сдвигами мембранного потенциала, приводящими к устойчивым разрядам потенциала действия с последующей сильной гиперполяризацией. Они обычно состоят из большой амплитуды короткого спайка (продолжительностью 50–100 мс), за которым обычно следует медленная волна (длительностью 200–500 мс) (de Curtis, Avoli, 2016), которая представляет собой гиперполяризацию (Lévesque et al., 2019). Важным свойством интериктальных разрядов как у пациентов, так и у экспериментальных животных является синхронизация, независимо от того, в каких условиях происходила их генерация (Ridler et al., 2018; Lévesque et al., 2019; Lévesque et al., 2020).
В то же время, несмотря на большой интерес к проблеме синхронизации, многие механизмы ее возникновения в коре остаются неисследованными. В задачу данной работы входила попытка выяснить, какие свойства коры определяют взаимосвязи между соседними нейронными сетями, которые отражаются в синхронизации интериктальных разрядов.
МЕТОДИКА
При всех манипуляциях с животными соблюдалась Директива 2010/63/EU о защите животных, используемых для экспериментальных и других научных целей. Опыты были проведены на 12 взрослых крысах линии Вистар. В соответствии с Директивой, в каждой серии было использовано минимальное количество животных, необходимых для получения статистически достоверных результатов. Протокол исследований утвержден этической комиссией Института высшей нервной деятельности и нейрофизиологии РАН. Животных содержали в условиях искусственного светового режима (день 8.00–20.00; ночь 20.00–8.00). Во всех экспериментах использовали взрослых животных в возрасте старше 3 месяцев.
Хирургическая процедура. Подробно операция на животных была изложена в предыдущей работе (Марченко, Зайченко, 2015). При проведении хирургической процедуры в качестве наркоза использовался хлоралгидрат из расчета 400 мг/кг. Во время хлоралгидратного наркоза активность мозга напоминает то, что наблюдается во время естественного сна (Ward-Flanagan et al., 2022), В процессе регистрации электрической активности наркоз добавлялся в количестве 5–10% от первоначальной дозы для поддержания животного в состоянии легкого наркотического сна. Над черепом удаляли кожу и затем над правым полушарием для регистрирующих электродов просверливали 3 или 4 отверстия диаметром 0.5 мм в передне-заднем направлении между брегмой и лямбдой таким образом, что расстояния между ними составляли 2 мм. Рядом с крайними отверстиями для электродов в кости просверливали отверстия 2 мм в диаметре для аппликации веществ – блокаторов ГАМК-А-рецепторов. Первая точка регистрации находилась в 1 мм от брегмы. Регистрация проводилась в первичной моторной, первичной сенсомоторной коре (Paxinos, Watson, 1998).
Регистрация электрической активности. Для длительной и непрерывной регистрации электрокортикограммы (ЭКоГ) в острых опытах использовали нихромовые электроды диаметром 150 мкм в изоляции, которые с помощью стереотаксиса вводили в отверстия в кости до твердой мозговой оболочки. Индифферентный электрод располагался на кости над лобной пазухой. Интериктальные разряды, представляющие собой первоначальный острый пик (спайк) длительностью несколько десятков миллисекунд и последующую медленную волну, вызывали аппликацией на поверхность коры пенициллина (Arnal-Real et al., 2021), разведенного в физиологическом растворе на одно или одновременно на оба отверстия в объеме 0.03–0.05 мл в необходимой концентрации до 1 000 000 ед. в 1 мл. Электрическую активность регистрировали до и после применения пенициллина. Регистрацию ЭКоГ крыс проводили практически непрерывно в течение всего эксперимента с помощью усилителя “НейроБиоЛаб” в диапазоне частот 1–70 Гц с подавлением артефактов 50 Гц сети электропитания. С использованием системы сбора данных NBL 140P-4 суммарная электрическая активность оцифровывалась с частотой дискредитации 100 кГц и накапливалась на жестком диске компьютера в формате abf с помощью программы AxoScope (Axon Instruments/ Molecular Devices).
Обработка данных. В течение каждого опыта последовательно визуально выбирались отрезки ЭКоГ длительностью 1 или 2 мин (в зависимости от характера активности), на которых вначале отсутствовала синхронизация между интериктальными разрядами в двух точках коры, а далее возникала синхронизация. С помощью программы Spike 2 электрическая активность переводилась в электронные таблицы. Определялись точки экстремума интериктальных спайков – самой нижней точки острого пика (рис. 1 (а) стрелки).
Рис. 1.
Метод определения временно́й задержки между возникновением пиков интериктальных разрядов, определение длительности медленной фазы (волны) интериктального разряда. (а) – определение временно́й задержки между возникновением интериктальных разрядов с помощью вертикальных курсоров 1, 2. (б) – определение длительности медленной волны на уровне половины амплитуды (горизонтальные курсоры 3, 4). (в) – отрезок ЭКоГ с несинхронными и синхронными интериктальными разрядами, (г) – одновременное возникновение интериктальных разрядов. Fig. 1. Method for determining the time delay between the occurrence of interictal discharge peaks, determining the duration of the slow phase (wave) of the interictal discharge. (а) – determination of the time delay between the occurrence of interictal discharges using vertical cursors. (б) – determination of the duration of the slow wave at the half amplitude level (horizontal cursors). (в) – section of ECoG with non-synchronous and synchronous interictal discharges, (г) – simultaneous occurrence of interictal discharges.
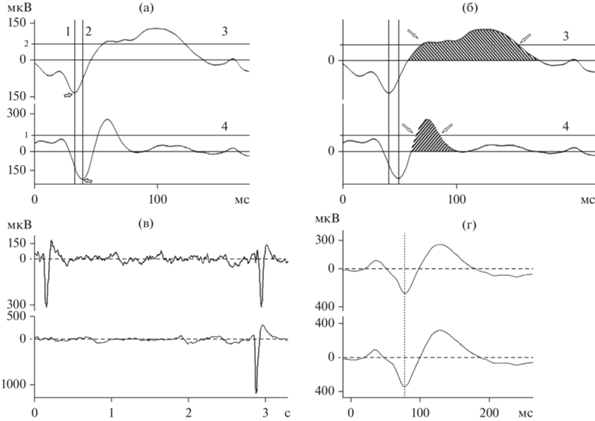
Временна́я задержка между моментами возникновения интериктальных разрядов в точках коры, находящихся на расстоянии 2 мм, определялась как временно́й интервал между точками экстремума первой фазы интериктальных разрядов с помощью вертикальных курсоров 1 и 2 (на рис. 1 (а)). Длительность медленной волны интериктального разряда, следующей после пика, (заштрихованные области на рис. 1 (б)), определялась в миллисекундах равной длине отрезка на середине амплитуды посредством горизонтальных курсоров 3 и 4 (на рис. 1 (б)) на пересечении горизонтальных курсоров с линией ЭКоГ (стрелки). Для каждой пары интериктальных разрядов определялось отношение длительности медленной волны интериктального разряда.
Статистическая обработка. С помощью программы статистического анализа Statistica 7 и Exel 10 проводилась обработка полученных данных. Рассчитывались средние величины временно́й задержки между моментами возникновения интериктального разряда, средние значения длительности медленной волны интериктального разряда для каждого опыта и распределение по всем опытам. Степень синхронизации между интериктальными разрядами в каждом временно́м отрезке длительностью от 1 до 2 мин определялась как стандартное отклонение временно̀й задержки между возникновением интериктальных спайков. Чем меньше величина разброса стандартного отклонения, тем сильнее синхронизация. Распределение значений исследованных параметров было проверено на нормальность по критерию Колмогорова–Смирнова (Basic Statistics, раздел Descriptive Statistics). Так как данные не соответствовали критерию нормальности, то для оценки различий между длительностями медленной волны интериктальных разрядов использовали непараметрический критерий Манна–Уитни (Nonparametric Statistics). Различия считали статистически значимыми при р < 0.05.
Модель обратных связей на программе Neuron. С помощью программы Neuron – симуляционной среды для моделирования отдельных нейронов и сетей (Carnevale, Hines, 2006) – была построена простая симуляционная модель популяции нейронов коры, состоящей из двух простых нейронных сетей, каждая из которых включала 4 возбудительных нейрона и 2 тормозных. Модель была построена на основании функциональных связей (Марченко, Зайченко, 2022). В каждой нейронной сети возбудительные нейроны были связаны между собой, а нейронные сети между собой были связаны обратными тормозными связями.
РЕЗУЛЬТАТЫ ИССЛЕДОВАНИЙ
После блокады ГАМК-А-рецепторов в коре происходит генерация интериктальных разрядов в разных участках коры. Необходимо отметить, что синхронизация возникает не сразу, а через несколько минут. В процессе регистрации активность интериктальных разрядов визуально можно было разделить на три этапа. Вначале эпилептиформные разряды возникают с задержкой в случайном порядке в разных точках коры. На первом этапе спайки в соседних участках коры возникают неодновременно. При этом происходит увеличение их амплитуды за счет одновременной активации элементов в локальной нейронной сети – явление локальной синхронизации. На втором этапе интериктальные разряды возникают как в случайном порядке, так и почти одновременно в соседних участках коры (рис. 1 (в)). На следующем этапе, после того как в одной точке коры возникает интериктальный разряд, в соседней точке коры происходит генерация интериктального разряда, или с небольшой задержкой, как на рис. 1 (а, б), или одновременно (рис. 1 (г)).
Длительность временно́й задержки. В условиях синхронизации длительность временной задержки между возникновением интериктальных разрядов в соседних точках коры составляла в среднем в разных опытах от 0 до 66 мс и сильно различалась у разных животных (рис. 2 (а)). При этом наблюдались два типа временны́х задержек. В некоторых экспериментах разряды возникали с задержкой от 0 до 2.7 мс (рис. 3 (б)), а в других опытах задержка составляла от 2.7 до 66 мс (рис. 2 (б)). Существенно, что в отсутствие синхронизации (случайное возникновение интериктальных разрядов) временна́я задержка между интериктальными разрядами была больше 70 мс и в некоторых случаях составляла несколько секунд.
Рис. 2.
Временна́я задержка между возникновением интериктальных разрядов и стандартное отклонение временно́й задержки. (а) – примеры распределения временны́х задержек в двухминутных отрезках в 3 опытах. (б) – распределение средних величин временной задержки в условиях синхронизации во всех опытах (12). (в) – изменение стандартного отклонения (степени синхронизации) во время проведения одного опыта. (г) – распределение величины стандартного отклонения по всем опытам в условиях синхронизации. Fig. 2. Time delay between the occurrence of interictal discharges and the standard deviation of the time delay. (а) – distribution of the time delays in two-minute intervals in three experiments. (б) – distribution of the mean values of the delays under synchronization conditions. (в) – change in the standard deviation (degree of synchronization) during the experiment. (г) – distribution of the value of the standard deviation over the experiments under synchronization conditions.
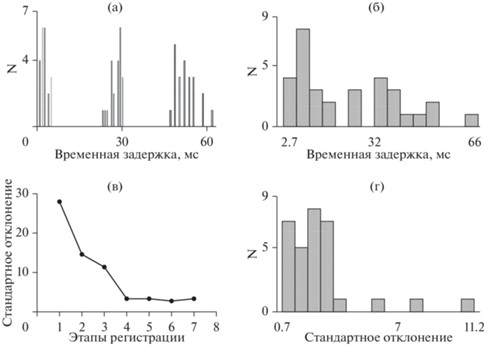
Рис. 3.
Параметры синхронизации при коротких временных задержках между возникновением интериктальных разрядов (до 3 мс). (а) – распределение значений стандартного отклонения (степени синхронизации). (б) – распределение величины временной задержки. (в) – отношение длительностей медленной волны интериктального разряда в 2 точках коры, расположенных на расстоянии 2 мм. (г) – гистограмма рассеивания значений отношений длительности медленной волны интериктального разряда в точке 1 (генератор) к длительности в точке 2 (ведомый) к временно́й задержке в этих точках коры в течение всего опыта от начала (точки в левом нижнем углу) до конца (точки вверху) одного опыта. (д) – то же, что и на (г), но для всех случаев с короткой временно́й задержкой по средним значениям. Fig. 3. Synchronization parameters for short time delays between the occurrence of interictal discharges. (а) – distribution of the standard deviation values (degree of synchronization). (б) – distribution of the time delay value. (в) – ratio of durations of slow wave interictal discharges in cortical points at a distance of 2 mm. (г) – histogram of scatter values of the ratio of the duration of the slow wave of interictal discharge in point 1 (generator) to the duration in point 2 (slave) to the time delay in these cortical points at the beginning and end of one experiment. (д) – the same as in (г), but for all experiments with a short time delay.
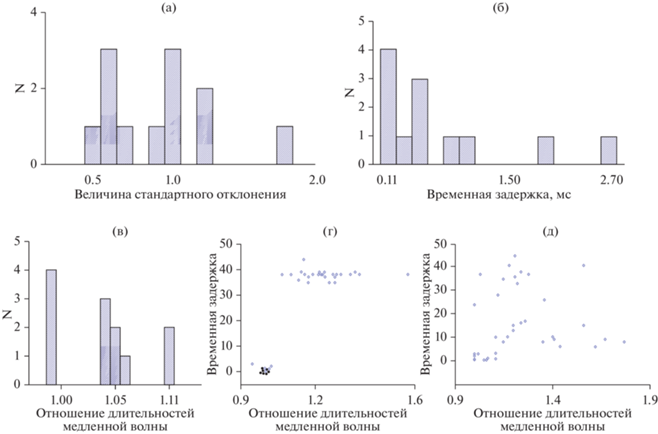
Синхронизация. Стандартное отклонение величины временно́й задержки между моментами возникновения интериктальных разрядов в точках коры, находящихся на расстоянии 2 мм, было выбрано в качестве количественного критерия синхронизации в отрезках длительностью до 2 минут. Этот показатель в индивидуальных опытах уменьшался от 1500 в период отсутствия синхронизации до минимального значения 0.7 (рис. 2 (г)), когда синхронизация была максимальной. Критерием полной синхронизации в отрезке ЭКоГ являлось наличие только пар интериктальных разрядов и отсутствие одиночных разрядов. Это совпадало с моментом, когда каждому интериктальному разряду в одной точке коры соответствовал интериктальный разряд в другой. Если же разряды возникали не по парам, то стандартное отклонение было значительно больше. Как видно на рис. 2 (в), в одном опыте в точке ноль (не представлена на рисунке) значение стандартного отклонения было 1215, 28 – в точке 1, и в точке 3 – 11.2. В точках 4, 5, 6, 7 величина стандартного отклонения временно́й задержки была минимальной (полная синхронизация) и незначительно колебалась в течение длительного времени, и на рис. 2 (в) это выглядит как плато.
Степень синхронизации (величина стандартного отклонения) на первом и на втором этапах составляла в среднем 488. Минимальное значение 14, максимальное 1583 (полное отсутствие синхронизации). На третьем этапе в условиях полной синхронизации среднее значение для всех опытов составляло 2.6, а диапазон значений, в котором наблюдалась сильная синхронизация, составлял от 0.7 до 11.2 (рис. 2 (г)).
Общий вход. В некоторых опытах были получены результаты, показывающие одинаковые параметры возникновения интериктальных разрядов. В этих случаях величины задержки были близки к нулевым значениям – интериктальные разряды возникали или одновременно, или с очень короткой задержкой, в среднем менее 2.7 мс (рис. 3 (б)). При этом степень синхронизации была минимальной – значения стандартного отклонения (мера рассеивания от средней) колебались от 0.5 до 1.7 (рис. 3 (а)). Важным свойством интериктальных разрядов, возникающих с очень короткой временно́й задержкой, являлась одинаковая длительность медленной волны интериктального разряда. Это хорошо показывает отношение длительности медленной волны в одной точке коры к другой, которое было около единицы, разница этих величин была близка к погрешности измерения (рис. 3 (в)). Необходимо отметить, что величина временно́й задержки между моментами возникновения интериктальных разрядов в соседних точках коры и отношение длительностей второй фазы, как правило, существенно увеличивалось в процессе регистрации в одном опыте (рис. 3 (г)). Это говорит о том, что если в начале регистрации интериктальные разряды в соседних нейронных сетях инициируются из одного источника, то со временем функция генератора переходит к одной из соседних нейронных сетей в результате изменения нейронных связей в коре.
Переход функции генератора к соседней нейронной сети влечет за собой изменение свойств медленной волны интериктальных разрядов. Отношение величин их длительностей у каждого животного (как правило) значительно меняется с увеличением временно́й задержки между возникновением интериктальных разрядов (рис. 3 (д)).
Свойства интериктальных разрядов в отсутствие синхронизации. Существенный интерес для нас представляли свойства интериктальных разрядов в ситуации, когда отсутствовала связь между соседними нейронными сетями или она была неустойчивой, когда интериктальные разряды возникли как отдельно, так и с большой задержкой. Определение степени синхронизации в этой ситуации (проведенное формально и служащее контролем для сравнения с периодом полной синхронизации) показало, что этот показатель (стандартное отклонение) находился в диапазоне от 14 до 1583 (рис. 4 (а)). Средние величины длительности медленной волны интериктального разряда в одной точке коры (1) находились в диапазоне от 23 до 55 мс, а в другой (2) от 29 до 51 мс (рис. 4 (б)), соответственно, и средние значения были одинаковы – 40 мс. Существенно, что с увеличением синхронизации длительность медленной волны существенно увеличивалась в каждом опыте, как в 1-й, так и во 2-й точках коры (рис. 4 (г)). Хотя через какое-то время этот показатель начинал уменьшаться.
Рис. 4.
Параметры синхронизации и длительности медленных фаз без синхронизации между интериктальными разрядами в 2 точках коры в 12 опытах. (а) – распределение величины стандартного отклонения временной задержки при отсутствии синхронизации. (б) – распределение длительностей медленной волны в точке 1 (генератор). (в) – распределение длительностей медленной волны в точке 2 (ведомый). (г) – изменение длительности медленной волны в течение эксперимента в 2 опытах (1, 2 и 3, 4 соответственно) в двух точках коры. Fig. 4. Parameters of synchronization and durations of slow phases without synchronization between interictal discharges in 2 cortical points in 12 experiments. (а) – distribution of the value of the standard deviation of the time delay in the absence of synchronization. (б) – distribution of slow wave durations at point 1 (oscillator). (в) – distribution of slow wave durations in point 2 (slave). (г) – distribution of slow wave durations during the experiment in 2 experiments (1, 2 and 3, 4, respectively) at two points of the cortex.
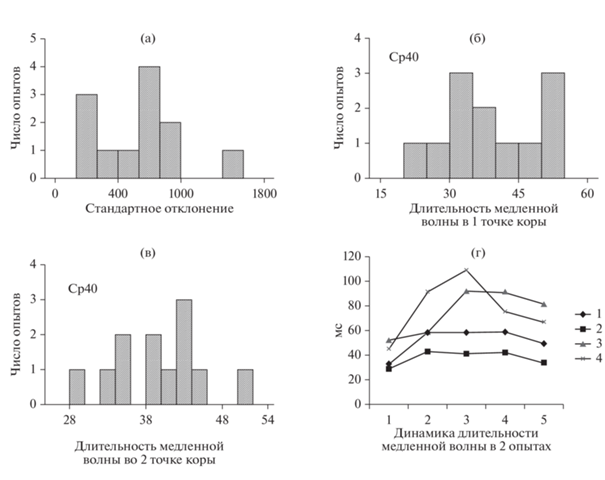
Длительность медленной волны интериктального разряда. В процессе регистрации происходило увеличение длительности медленной волны интериктального разряда как в одной точке коры, так и в другой, находящейся на расстоянии 2 мм. Это наглядно показывает отношение длительности медленной волны интериктального разряда в условиях синхронизации к ее длительности на этапе отсутствия синхронизации как в 1-й, так и во 2-й точках коры. В некоторых опытах длительность медленной волны при синхронизации была почти в 3 раза длиннее, чем при отсутствии синхронизации (рис. 5 (а, б)). Так, в условиях синхронизации максимальная средняя длительность медленной волны интериктального разряда для каждого опыта была длиннее в 1.43–2.77 раз в одной точке коры, в среднем в 1.78 раз (рис. 5 (а)), в другой – в 1.0–2.43 раз, в среднем в 1.63 раз (рис. 5 (б)), по сравнению с длительностью на начальном этапе при отсутствии синхронизации. Длительности медленных волн достоверно различались по критерию Mann–Whitney U test с р = 0.002 и р = 0.003 в одной и другой точке коры соответственно. Самым важным результатом работы является то, что в условиях полной синхронизации длительность медленной волны в точке коры, в которой интериктальный разряд генерируется первым, существенно длиннее по сравнению с точкой, в которой разряд возникал с задержкой. Как показали наши результаты, отношение длительности медленной волны в точке генерации к длительности во второй точке было от 1.0 до 1.77 (рис. 5 (в)) и достоверно различалось с р < 0.0001. Особенно наглядно это видно на диаграмме рассеивания, на которой представлено сравнение этих показателей в одном опыте (рис. 5 (г)).
Рис. 5.
Параметры длительности медленной фазы интериктального разряда. (а) – отношение средних значений длительности медленных фаз в каждом опыте в условиях сильной синхронизации и при еe отсутствии (контроль) в 1-й точке коры (генератор). (б) – то же, что и на (а), но во 2-й точке. (в) – отношение средних значений длительности медленных фаз в условиях сильной синхронизации между двумя точками коры во всех опытах. (г) – гистограмма рассеивания длительностей медленной фазы в точке 1 (ордината) и точке 2 (абсцисса) коры в одном опыте. Fig. 5. Parameters of the slow phase duration of the interictal discharge. (а) – ratio of average values of slow phase duration in each experiment under conditions of strong synchronization and in its absence in the 1st point of the cortex (generator). (б) – the same as in (а), but in the 2nd point. (в) – ratio of average values of slow phases duration in conditions of strong synchronization between two points of the cortex in all experiments. (г) – histogram of dispersion of slow phase durations at point 1 (ordinate) and point 2 (abscissa) of the cortex in one experiment.
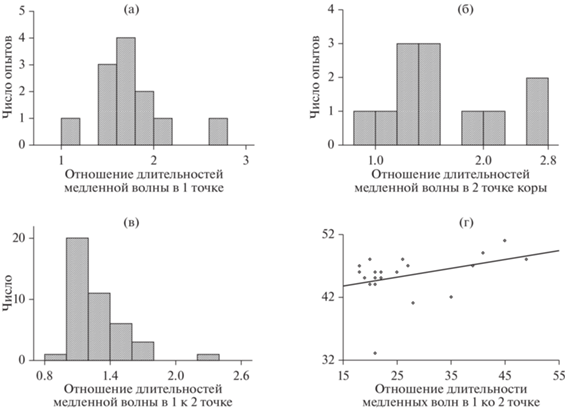
Исследования на симуляционной модели в среде “NEURON” роли обратных тормозных связей в синхронизации нейронной активности показали, что в процессе работы модели спайки генерировались в обеих нейронных сетях. Синхронизация между сетями происходила только тогда, когда активировалась тормозная обратная связь между ними. В отсутствие обратной тормозной связи синхронизация между спайками не возникала.
ОБСУЖДЕНИЕ РЕЗУЛЬТАТОВ
Основными результатами нашей работы являются:
1. После нанесения блокатора ГАМК-А-рецепторов пенициллина по мере усиления синхронизации между интериктальными разрядами происходит удлинение медленной волны во всех точках коры. Существенно, что в тех опытах, в которых наблюдалась временная задержка между возникновением интериктальных разрядов, длительность медленной волны в интериктальном разряде, возникшем первым (генератор), была достоверно длиннее, чем в соседнем.
2. Если задержка была минимальной, то длительности медленной волны были практически одинаковыми (контроль).
3. Известно на основании работ, сделанных в предпоследней декаде прошлого века, что после блокады ГАМК-А-рецепторов основным действующим лицом гиперполяризации являются ГАМК- Б-рецепторы. Тормозные ГАМК-ергические нейроные сети являются основными участниками интериктальных спайков в СА1 (Muldoon et al., 2015). В исследованиях на срезах гиппокампа с использованием блокатора ГАМК-А-рецепторов пенициллина было определено, что послегиперполяризация нейрона содержала компоненты, определяемые ГАМК-Б-рецепторами и кальций-зависимыми калиевыми токами (Domann et al., 1991). В моторной коре in vivo с применением пенициллина медленная послегиперполяризация включала в себя медленный кальций-зависимый калиевый ток и синаптический ГАМК-Б-ответ. После применения ЭГТА (EGTA) послегиперполяризация в клетках моторной коры была немного короче, чем в контрольных условиях. Эти эксперименты предполагают, что медленная послегиперполяризация частично определяется кальций-зависимыми калиевыми токами (Witte, 1994). Было показано, что во многих областях мозга поздняя фаза гиперполяризации отражает постсинаптическую активацию ГАМК-Б-рецепторов. Медленный ТПСП имеет свойства, сходные с ответом, вызванным активацией ГАМК-Б-рецепторов. ГАМК-Б-рецепторы опосредуют медленный ТПСП, который блокируется антагонистом ГАМК-Б-рецепторов факлофеном (Nicoll et al., 1990). Применение антагониста ГАМК-Б-рецепторов CGP55845A блокировало все моносинаптические поздние IPSP в пирамидных нейронах среза гиппокампа (Pham et al., 1998). Такие же исследования проводились на коре. В коре интериктальные спайки завершались большим постпотенциалом, блокированным внеклеточным применением факлофена и саклофена. В контрольных условиях, до введения бикукуллина, саклофен и факлофен также полностью отменяли поздний тормозной постсинаптический потенциал, опосредованный ГАМК-Б-рецепторами (de Curtis et al., 1999). На срезе гиппокампа мышей при применении бикукуллина максимальное уменьшение длительности гиперполяризации в гранулярных клетках гиппокампа происходит при применении блокатора ГАМК-Б-рецепторов CGP 55845 (Mircheva et al., 2010).
Детальный анализ данных об обратных тормозных анатомических и функциональных связях в коре и роли ГАМК-Б-рецепторов в механизмах синхронизации интерикальных разрядов дан на основании экспериментальных и теоретических работ (Марченко, Зайченко, 2022). Самым вероятным механизмом синхронизации интерикальных разрядов в условиях блокады ГАМК-А-рецепторов являются тормозные обратные связи посредством ГАМК-Б-рецепторов.
Результаты нашей работы также показали, что в условиях блокады ГАМК-А-рецепторов существует два типа синхронизации интериктальных разрядов в коре мозга крыс, в которых принимает непосредственное участие торможение посредством ГАМК-Б-рецепторов. Исходя из литературных данных, известных в настоящее время (Марченко, Зайченко, 2022), можно сделать вывод, что механизмы синхронизации в нейронных сетях неокортекса обеспечиваются коротким временны́м окном интеграции, которое создается и обратным торможением.
В некоторых наших опытах в условиях полной синхронизации интериктальные разряды возникали одновременно (с задержкой 0 мс) или при минимальной задержке не более 3 миллисекунд в точках коры, расположенных на расстоянии 2 мм. Характерно, что длительности медленной волны интериктального разряда у них были почти одинаковые. Короткая временная задержка между разрядами в соседних точках коры наиболее вероятно связана с тем, что пространственная синхронизация нейронов в разных областях коры может создаваться, когда на общий возбуждающий вход пирамидных нейронов одновременно подаются сигналы от сенсорных структур или других областей коры, или от таламуса, через активацию ГАМК-Б-рецепторов и создание временно́го окна интеграции (Chittajallu et al., 2013). Таким же образом создается синхронизация спайк-волны разрядов в таламокортикальных нейронных сетях с помощью обратных связей и созданием временны́х окон интеграции (Terlau et al., 2020).
Синхронизация активности соседних возбудительных клеток и интернейронов может происходить в результате стимуляции общего входа. Действительно, стимуляция пирамидных клеток слоя 2/3 вызывала синхронные ВПСП с постоянной латентностью в обеих клетках в паре пирамида/интернейрон, имеющих обратные связи друг с другом. Причем связи между пирамидными и тормозными клетками более эффективны при наличии обратной связи (Otsuka, Kawaguchi, 2009). Отметим, что длительности медленной волны интериктального разряда в нашей работе в двух соседних точках коры были или одинаковые, или близкие по величине. Можно предположить, что при этом действовали обратные связи между пирамидными нейронами и интернейронами, как было показано в экспериментальных работах (Otsuka, Kawaguchi, 2009; Terlau et al., 2020).
Обратное торможение является обязательным параметром синхронизации интериктальных разрядов. Наше исследование показало, что в период, когда интериктальные разряды возникали спонтанно, то есть не были синхронизированы в соседних точках коры, длительность их медленной волны (торможения) была одинаковой и короткой. В условиях максимальной синхронизации интериктальные разряды сначала возникали в одной точке коры (генератор), а затем в соседних точках с задержкой. При этом наблюдалось увеличение длительности медленной волны интериктального разряда в точке коры, в которой разряд возник раньше (генератор) по сравнению с соседней точкой. Можно предположить с большой долей уверенности, что это происходит вследствие действия обратных тормозных связей между этими нейронными сетями коры таким же образом, как ранее было показано в экспериментальных работах. Так, синхронизация пирамидных нейронов осуществлялась посредством обратных тормозных связей при стимуляции интернейронов (Hilscher et al., 2017). Посредством обратного торможения разных типов интернейронов в условиях наибольшей синхронизации увеличение длительности торможения происходит вследствие того, что на медленную волну интериктального разряда генератора, которая является тормозной фазой, добавляется торможение, инициируемое от соседней точки коры по обратным связям (Morishima et al., 2017).
Предполагалось, что наиболее вероятным механизмом синхронизации интериктальных спайков в нейронных сетях коры является создание с помощью торможения узкого временнόго окна, в течение которого возможна активность нейронов (Muldoon et al., 2015) и, соответственно, их интеграция, отражением которой и является синхронизация. Временно́е окно синхронизации создается за счет обратных тормозных связей соматостатиновых и парвальбуминовых интернейронов неокортекса (Jang et al., 2020) и клеток Мартинотти в гиппокампе (Simonnet et al., 2017).
Как показали наши опыты, при случайном возникновении интериктальных разрядов сразу после блока ГАМК-А-рецепторов (отсутствие синхронизации) длительность второй фазы интериктального разряда была короткой и отличалась в разных точках коры. Необходимо отметить, что временно́е окно в наших опытах создавалось постепенно с момента блока ГАМК-А-рецепторов в течение нескольких минут. В локальных нейронных сетях это отражалось в увеличении амплитуды интериктального спайка (возбудительной фазы) интериктального разряда, и таким образом наблюдалась локальная синхронизация. В то же время происходило создание временно́го окна в удаленных нейронных сетях и усиление степени пространственной синхронизации.
Результаты, полученные на симуляционной модели, также показали, что для синхронизации активности в простых нейронных сетях необходима обратная тормозная связь.
ЗАКЛЮЧЕНИЕ
Увеличение длительности медленной волны интериктального разряда (тормозной фазы) в условиях усиления синхронизации и большая ее длительность в точке генерации по сравнению с длительностью в соседней точке, а также возникновение интериктального разряда с существенной временно́й задержкой в условиях полной синхронизации в соседней точке свидетельствуют об участии обратного торможения в механизмах синхронизации в нейронных сетях коры крыс.
Список литературы
Марченко В.Г., Зайченко М.И. Динамика пространственной синхронизации эпилептиформных разрядов в неокортексе крыс. Журн. высш. нерв. деят. им. И.П. Павлова. 2015. 65 (1): 113–125.
Марченко В.Г., Рысакова М.П., Зайченко М.И. Распространение внутренних электрических полей как возможный механизм синхронизации интериктальных спайков в неокортексе крыс. Журн. высш. нерв. деят. им. И.П. Павлова. 2018. 68 (2): 250–262.
Марченко В.Г., Зайченко М.И. ГАМК Б торможение через обратные связи участвует в синхронизации интериктальных спайков в коре. Успехи физиологических наук. 2022. 53 (1): 1–24.
Amakhin D.V., Ergina J.L., Chizhov A.V., Zaitsev A.V. Synaptic conductances during interictal discharges in pyramidal neurons of rat entorhinal cortex front. Cell. Neurosci. 2016. 10: 233.
Bink H., Sedigh-Sarvestani M., Fernandez-Lamo I., Kini L., Ung H., Kuzum D., Vitale F., Litt B., Contreras D. Spatiotemporal evolution of focal epileptiform activity from surface and laminar field recordings in cat neocortex. J. Neurophysiol. 2018. 119 (6): 2068–2081.
Chittajallu R., Pelkey K.A., McBain C.J. Neurogliaform cells dynamically regulate somatosensory integration via synapse-specific modulation. Nat. Neurosci. 2013. 16 (1): 13–15.
Connors B.W. Synchrony and so much more: Diverse roles for electrical synapses in neural circuits. Dev Neurobiol. 2017. 77 (5): 610–624.
de Curtis M., Avoli M. GABAergic networks jump-start focal seizures. Epilepsia. 2016. 57 (5): 679–687.
de Curtis M., Radici C., Forti M. Cellular mechanisms underlying spontaneous interictal spikes in an acute model of focal cortical epileptogenesis. Neuroscience. 1999. 88 (1): 107–117.
Ding L., Satish S., Zhou C., Gallagher M.J. Cortical activation in generalized seizures. Epilepsia. 2019. 60 (9): 1932–1941.
Domann R., Dorn T., Witte O.W. Afterpotentials following penicillin-induced paroxysmal depolarizations in hippocampal pyramidal cells in vitro. Pfliigers Arch. 1991. 417: 469–478.
Gerrard L.B., Tantirigama M.L.S., Bekkers J.M. Pre- and Postsynaptic Activation of GABA B Receptors Modulates Principal Cell Excitation in the Piriform Cortex. Front Cell Neurosci. 2018. 12: 28.
Gibson J.R., Beierlein M., Connors B.W. Functional properties of electrical synapses between inhibitory interneurons of neocortical layer 4. J. Neurophysiol. 2005. 93 (1): 467–80.
Gray C.M., Singer W. Stimulus-specific neuronal oscillations in orientation columns of cat visual cortex. Proc. Natl. Acad. Sci. U S A. 1989. 86 (5): 1698–702.
Hilscher M.M., Leão R.N., Edwards S.J., Leão K.E., Kullander K. Chrna2-Martinotti cells synchronize layer 5 type a pyramidal cells via rebound excitation. PLoS Biol. 2017. 15 (2): e2001392.
Isaacson J.S., Scanziani M. How inhibition shapes cortical activity. Neuron. 2011. 72 (2): 231–243.
Jang H.J., Chung H., Rowland J.M., Richards B.A., Kohl M.M., Kwag J. Distinct roles of parvalbumin and somatostatin interneurons in gating the synchronization of spike times in the neocortex. Sci. Adv. 2020. 6 (17): eaay5333.
Jurado-Parras M.T., Delgado-García J.M., Sánchez-Campusano R., Gassmann M., Bettler B., Gruart A. Presynaptic GABAB receptors regulate hippocampal synapses during associative learning in behaving mice. PLoS One. 2016. 11 (2): e0148800.
Khazipov R. GABAergic Synchronization in Epilepsy. Cold Spring Harb Perspect Med. 2016. 6 (2): a022764.
Knoblich U., Huang L., Zeng H., Li L. Neuronal cell-subtype specificity of neural synchronization in mouse primary visual cortex. Nat. Commun. 2019. 10 (1): 2533.
Köhling R., D’Antuono M., Benini R., de Guzman P., Avoli M. Hypersynchronous ictal onset in the perirhinal cortex results from dynamic weakening in inhibition. Neurobiol. Dis. 2016. 87: 1–10.
Knowles W.D., Schneiderman J.H., Wheal H.V., Stafstrom C.E., Schwartzkroin P.A. Hyperpolarizing potentials in guinea pig hippocampal CA3 neurons. Cell Mol Neurobiol. 1984. 4 (3): 207–230.
Kubista H., Boehm S., Hotka M. The paroxysmal depolarization shift: reconsidering its role in epilepsy. Epileptogenesis and Beyond. Int. J. Mol. Sci. 2019. 20 (3): 577.
Lévesque M., Biagini G., Avoli M. Neurosteroids and Focal Epileptic Disorders. Int. J. Mol. Sci. 2020. 21 (24): 9391.
Lévesque M., Ragsdale D., Avoli M. Evolving mechanistic concepts of epileptiform synchronization and their relevance in curing focal epileptic disorders Curr. Neuropharmacol. 2019. 17 (9): 830–842.
Li Q., Li Q.-Q., Jia J.-N., Liu Z.-Q., Zhou H.-H., Mao X.-Y. Targeting gap junction in epilepsy: Perspectives and challenges.Biomed Pharmacother. 2019. 109: 57–65.
Mircheva Y., Peralta M.R. 3rd, Tóth K. Interplay of entorhinal input and local inhibitory network in the hippocampus at the origin of slow inhibition in granule cells. J. Neurosci. 2019. 39 (33): 6399–6413.
Morishima M., Kobayashi K., Kato S., Kobayashi K., Kawaguchi Y. Segregated excitatory-inhibitory recurrent subnetworks in layer 5 of the rat frontal cortex. Cereb. Cortex. 2017. 27 (12): 5846–5857.
Muldoon S.F., Villette V., Tressard T., Malvache A., Reichinnek S., Bartolomei F., Cossart R. GABAergic inhibition shapes interictal dynamics in awake epileptic mice. Brain. 2015. 138 (Pt 10): 2875–2890.
Nicoll R.A., Malenka R.C., Kauer J.A. Functional comparison of neurotransmitter receptor subtypes in mammalian central nervous system. Physiol Rev. 1990. 70 (2): 513–565.
Otsuka T., Kawaguchi Y. Cortical inhibitory cell types differentially form intralaminar and interlaminar subnetworks with excitatory neurons. J. Neurosci. 2009. 29 (34): 10533-40.
Paxinos G., Watson C. The Rat Brain in Stereotaxic Coordinates. San Diego: Academic Press, 1998.
Pham T.M., Nurse S., Lacaille J.C. Distinct GABAB actions via synaptic and extrasynaptic receptors in rat hippocampus in vitro. J. Neurophysiol. 1998. 80 (1): 297–308.
Ridler T., Matthews P., Phillips K.G., Randall A.D., Brown J.T. Initiation and slow propagation of epileptiform activity from ventral to dorsal medial entorhinal cortex is constrained by an inhibitory gradient. J. Physiol. 2018. 596 (11): 2251–2266.
Serrats J., Cunningham M. O., Davies C. H. GABA B receptor modulation-to B or not to be B a pro-cognitive medicine? Curr. Opin. Pharmacol. 2017. 35: 125–132.
Simonnet J., Nassar M., Stella F., Cohen I., Mathon B., Boccara C. N., Miles R., Fricker D. Activity dependent feedback inhibition may maintain head direction signals in mouse presubiculum. Nat. Commun. 2017. 8: 16032.
Terlau J., Yang J.-W., Khastkhodaei Z., Seidenbecher T., Luhmann H.J., Pape H.-C., Lüttjohann A. Spike-wave discharges in absence epilepsy: segregation of electrographic components reveals distinct pathways of seizure activity. J. Physiol. 2020. 598 (12): 2397–2414.
Trevelyan A.J. Do cortical circuits need protecting from themselves? Trends Neurosci. 2016. 39 (8): 502–511.
Wang Y., Neubauer F.B., Lüscher H.R., Thurley K. GABAB receptor-dependent modulation of network activity in the rat prefrontal cortex in vitro. Eur. J. Neurosci. 2010. 31 (9): 1582–1594.
Wang G., Wu X. The potential antiepileptogenic effect of neuronal Cx36 gap junction channel blockage. Transl. Neurosci. 2021. 12(1): 46–51.
Witte O.W. Afterpotentials of penicillin-induced epileptiform neuronal discharges in the motor cortex of the rat in vivo. Epilepsy Res. 1994. 18 (1): 43–55.
Yekhlef L., Breschi G.L., Lagostena L., Russo G., Taverna S. Selective activation of parvalbumin- or somatostatin-expressing interneurons triggers epileptic seizure like activity in mouse medial entorhinal cortex. J. Neurophysiol. 2015. 113 (5): 1616–30.
Zheng Yu., Zhang K., Dong L., Tian C. Study on the mechanism of high-frequency stimulation inhibiting low-Mg 2+-induced epileptiform discharges in juvenile rat hippocampal slices.Brain Res Bull. 2020. 165: 1–13.
Дополнительные материалы отсутствуют.
Инструменты
Журнал высшей нервной деятельности им. И.П. Павлова