Коллоидный журнал, 2023, T. 85, № 6, стр. 781-794
Нековалентная стабилизация водорастворимого фталоцианината цинка в гидрозоле оксида графена
А. Г. Нугманова 1, А. И. Горшкова 2, А. В. Ягодин 1, А. А. Аверин 1, М. А. Калинина 1, *
1 Институт физической химии и электрохимии им. А.Н. Фрумкина РАН
119071 Москва, Ленинский просп. 31, корп. 4, Россия
2 Московский государственный университет им. М.В. Ломоносова, факультет наук о материалах
119991 Москва,
Ленинские Горы 1, стр. 73, Россия
* E-mail: kalinina@phyche.ac.ru
Поступила в редакцию 07.08.2023
После доработки 18.09.2023
Принята к публикации 18.09.2023
- EDN: XTLVXB
- DOI: 10.31857/S0023291223600700
Аннотация
В данной работе была изучена возможность стабилизации 2,3,9,10,16,17,23,24-окта[(3,5-бискарбоксилат натрия)фенокси]фталоцианината цинка(II) (ZnPc16) путем его гибридизации с поверхностью листов оксида графена (ОГ) за счет дисперсионных или координационных взаимодействий с фрагментами углеродного каркаса в объеме гидрозолей ОГ. С помощью сочетания физико-химических методов анализа (СЭМ, флуоресцентная микроскопия, рентгеновская порошковая дифракция, КР-спектроскопия) была подтверждена интеграция ZnPc16 с нанолистами ОГ и изучены морфология и структура полученных гибридных материалов. С помощью спектроскопии электронного поглощения установлено, что, независимо от метода гибридизации, связывание макроцикла с неорганической частицей приводит к увеличению устойчивости ZnPc16 в водной среде под действием видимого света. На основе анализа данных спектральных кинетических исследований показано, что, в отличие от системы, полученной путем прямой интеграции ZnPc16 и ОГ, гибридный материал, сформированный за счет координационных связей между компонентами с использованием ацетата цинка (Zn(OAc)2) в качестве связующего металлокластера, способен проявлять фотокаталитические свойства в окислительной фотодеструкции ряда модельных органических субстратов-поллютантов (родамина 6G, 1,5-дигидроксинафталина, 1,4-нитрофенола). Предложенный коллоидно-химический подход к стабилизации фотоактивных водорастворимых фталоцианинатов потенциально позволяет увеличивать их устойчивость к фотоиндуцированному самоокислению и может быть адаптирован для различных производных тетрапиррольных соединений, обладающих фотосенсибилизирующими свойствами.
ВВЕДЕНИЕ
Современный этап развития химической науки сфокусирован на интенсивном поиске новых технологий и материалов для химической промышленности, которые могли бы сократить потребление ископаемых энергоресурсов, а также снизить антропогенную нагрузку на окружающую среду [1]. Одно из наиболее перспективных направлений для достижения этих целей связано с разработкой материалов для фотокатализа, основанного на преобразовании энергии света, прежде всего, видимого солнечного спектра, в химическую работу как по образованию связей и синтезу новых соединений, так и для фотохимической дезактивации различных органических веществ [2]. К настоящему времени наиболее разработаны подходы к гомогенному фотокатализу, в котором используются полностью сольватированные соединения-фотокатализаторы в молекулярной форме [3, 4]. Как правило, в качестве таких соединений используются тетрапирролы и их производные, триазины, сопряженные полимеры и др. [5–9], которые отличаются высокой эффективностью и селективностью. В то же время существует целый ряд особенностей гомогенного фотокатализа, которые ограничивают его широкое применение. В частности, молекулярные фотокатализаторы, в особенности наиболее эффективные из них, неустойчивы и подвергаются самоотравлению во время фотохимической реакции, их трудно выделить из реакционной среды и восстановить их свойства, поэтому молекулярные фотокатализаторы часто сами становятся загрязнителями окружающей среды [10]. Эти ограничения могут быть частично или полностью сняты в гетерогенном фотокатализе, протекающем на поверхности дисперсных материалов, что способствует увеличению как экологической чистоты процесса, так и его экономической целесообразности [11]. Поскольку эффективность работы гетерогенного фотокатализатора прямо зависит от площади его поверхности, и большинство таких материалов представляют собой микро- или нанодисперсные системы, то их разработка является одной их актуальных задач современной коллоидной химии.
Твердые фотокатализаторы представляют собой материалы, обладающие высокой фотохимической активностью за счет способности к генерации и разделению фотоиндуцированного заряда [12]. В основном в качестве дисперсных фотокатализаторов используются различные неорганические полупроводники, такие как TiO2, ZnO, CdS, g-C3N4, оксид графена и др. [13–16]. Такие системы обладают механической, химической и фотохимической устойчивостью и легко поддаются выделению и реактивации. В то же время по своим фотокаталитическим свойствам подавляющее большинство неорганических твердых фотокатализаторов существенно уступает органическим молекулярным [17–19]. В связи с этим в последнее десятилетие активно разрабатываются подходы к получению гибридных материалов, которые способны обеспечить необходимую комбинацию структурных и фотокаталитических свойств за счет объединения фотоактивных органических и неорганических компонентов в различных сочетаниях [20–23]. Особый интерес представляет интеграция неорганических матриц с тетрапиррольными соединениями, такими как порфирины и фталоцианины [5, 10, 24, 25], обладающими целым рядом уникальных свойств: высокой степенью экстинкции, способностью поглощать свет в широком диапазоне длин волн, включая видимый и ближний ИК-диапазоны, возможность модификации различными функциональными группами, что позволяет получать соединения с различными каталитическими свойствами и улучшенной селективностью и эффективностью, а также низкой токсичностью относительно других молекулярных фотосенсибилизаторов [10, 25, 26]. По сравнению с порфиринами, фталоцианины и их комплексы более доступны, их мировое промышленное производство измеряется тысячами тонн для самых различных областей применения (фотодинамическая терапия [27], светостойкие зеленые и голубые красители и пигменты [28], оптические материалы для лазерных дисков и т.д. [20]). Фталоцианинаты различного строения используются в гомогенном фотокатализе, прежде всего, окислительной фотодеструкции за счет своей способности к выделению синглетного кислорода [29], в то же время именно она может приводить к быстрому самоотравлению даже у соединений с устойчивой химической структурой и провоцировать распад фталоцианинатов в процессе фотохимического окисления. Кроме того, большинство производных фталоцианинов нерастворимы в воде, что ограничивает их использование, поскольку неводные растворители в основном не отвечают критериям “зеленой химии”.
Интеграция фталоцианинатов с неорганическими матрицами потенциально позволяет решить обе проблемы, одновременно обеспечив стабилизацию и применимость этих фотосенсибилизаторов в водной среде. Гибридизация фталоцианинатов с поверхностью может осуществляться как с помощью боковых функциональных заместителей, например, за счет ковалентной сшивки с предварительно функционализированными частицами, а также ароматических или дисперсионных взаимодействий электронной системы макроцикла с поверхностью неорганической частицы [30–32]. К сожалению, до настоящего времени все попытки осуществить гибридизацию фталоцианинатов с наночастицами неорганических полупроводников за счет таких взаимодействий [31, 33, 34], хотя и способствовали увеличению устойчивости фталоцианиновых фотосенсибилизаторов в реакционной среде, сопровождались относительным снижением фотоокислительной активности макроциклического соединения вследствие тушения возбужденного триплетного состояния фталоцианина, обеспечивающего генерацию синглетного кислорода [33, 34].
Эта проблема может быть решена за счет предотвращения сильных непосредственных взаимодействий макроцикла с поверхностью при гибридизации, например, путем супрамолекулярной сборки упорядоченных гибридов с образованием пространственно-направленных координационных связей между неорганической матрицей и фотосенсибилизатором.
В качестве неорганической матрицы для такой гибридизации особый интерес представляет оксид графена (ОГ), который является окисленным производным графена – двумерного материала, состоящего из sp2-сопряженных атомов углерода [35]. Оксид графена обладает оптической прозрачностью, относительно низким уровнем Ферми, а также содержит функциональные группы (карбоксильные, эпоксидные, гидроксильные), способныe к образованию связей различных типов [16, 36, 37]. При взаимодействии с органическим фотосенсибилизатором ОГ может действовать как электронный проводник, способствуя миграции фотоиндуцированных носителей заряда [38], что, в свою очередь, приводит к повышению совокупной фотокаталитической активности гибрида. Ранее нами на примере тетразамещенных порфиринатов цинка(II) было показано, что гибридизация таких тетрапиррольных соединений с ОГ может осуществляться за счет образования координационных связей с металлокластером (ацетатом цинка), связывающим карбоксильные или пиридильные функциональные группы порфирината с карбоксильными группами на поверхности нанолистов ОГ [39, 40]. В отличие от гибридов порфиринатов аналогичного строения, полученных за счет ароматического стекинга между электронными системами макроцикла и углеродного каркаса, в таких системах благодаря супрамолекулярной организации порфиринатов на поверхности ОГ они сохраняют свою способность к генерации синглетного кислорода с одновременным повышением устойчивости к самоокислению [41]. В то же время до настоящего времени не было предпринято аналогичных попыток интеграции фталоцианинатов и ОГ за счет координационных взаимодействий.
В данной работе впервые изучена возможность гибридизации водорастворимого цинкового комплекса фталоцианина (2,3,9,10,16,17,23,24-окта[(3,5-бискарбоксилат натрия)фенокси]фталоцианината цинка(II)) [27] с нанолистами ОГ в объеме водных золей. Функционализация макроцикла 16-ю карбоксильными группами позволяет не только обеспечить его растворимость в воде, но и предотвращать сильные ароматические (стекинговые) взаимодействия между макроциклическим фрагментом молекулы ZnPc16 и sp2-системой ОГ за счет стерического эффекта заместителей. Ранее было показано, что ZnPc16 в водных растворах при облучении светом с длиной волны 680 нм способен к эффективной генерации синглетного кислорода как наиболее активной формы фотоиндуцированных кислород-содержащих интермедиатов [27]. В этой работе получены гибридные дисперсные системы на основе ZnPc16 и ОГ с использованием ацетата цинка (Zn(OAc)2) в качестве связующего металлокластера. Для оценки влияния типа взаимодействий между органическим и неорганическим компонентами на свойства макроцикла проведен сравнительный анализ устойчивости и активности таких систем в фотоокислительной деструкции ряда молекулярных субстратов (родамина 6G (Rh6G), 1,5-дигидроксинафталина (ДГН) и 1,4-нитрофенола (НФ)) по отношению к раствору фталоцианината и гибридной системе ZnPc16-ОГ, полученной в отсутствие соли металла.
ЭКСПЕРИМЕНТАЛЬНАЯ ЧАСТЬ
Материалы и методы
Воду предварительно очищали на аквадистилляторе ДЭ-10М и затем деионизировали с помощью деионизатора “Водолей” для получения деионизированной воды. Остальные реагенты и растворители – персульфат калия (K2S2O8, Sigma-Aldrich, >99%), оксид фосфора(V) (P2O5, Sigma-Aldrich, >98%), серная кислота (H2SO4, Реахим, ХЧ), соляная кислота (HCl, Реахим, ХЧ), перекись водорода Н2О2 (30 мас. %, Реахим, ХЧ), графитовая пудра (Графенокс, GNP 350), дигидрат ацетата цинка(II) (Zn(CH3COO)2∙2H2O, Sigma-Aldrich, ≥98%), 1,5-дигидроксинафталин (ДГН, Sigma-Aldrich, ≥97%), родамин 6G (Rh6G, Sigma-Aldrich, ≥99%), 1,4-нитрофенол (НФ, Sigma-Aldrich, ≥99%) были использованы без дополнительной очистки.
Взвешивание проводилось на аналитических весах Ohaus AdventurerPro. Для измельчения листов ОГ использовали ультразвуковой гомогенизатор VCX-750-220 с титановым зондом диаметром 13 мм. Состояние поверхности и электронная структура оксида графена были исследованы методом рентгеновской фотоэлектронной спектроскопии (РФЭС) (система сверхвысокого вакуума для поверхностного анализа Kratos AXIS UltraDLD) с использованием излучения Al K-alpha (1486 эВ) в качестве зонда. Энергия пропускания – 15 эВ, шаг съемки опорного спектра – 1 эВ, спектров С1s, O1s и Si2p – 0.1 эВ. Обработка спектров проводилась с использованием пакета CasaXPS. Спектры корректировались по положению sp2 линии углерода (284.6 эВ). Разложение проводилось на суперпозицию функций Гаусса и Лоренца в пропорции 50/50% (GL (50)), производилось вычитание фона по Ширли (Shirley).
Методом атомно-силовой микроскопии (АСМ) были получены АСМ-изображения с использованием сканирующего зондового микроскопа SOLVER P47-PRO (NT-MDT). Были использованы кремниевые зонды с высоким разрешением АСМ серии NSG01 (NT-MDT). Измерения проводились в полуконтактном режиме с амплитудой колебаний зонда “свободного воздуха” от 20 до 25 нм (пик-пик).
Для термической обработки образцов гибридных материалов использовали сушильный шкаф ЭКРОС ПЭ-4610. Для сушки образцов использовали эксикатор с мембранным вакуумным насосом KNF N 816-3 KT-18 и лиофильную сушилку ERSTEVAK серии EV-DF-SA.
Методом сканирующей электронной микроскопии (СЭМ) были получены СЭМ-изображения с использованием рабочей станции Carl Zeiss NVision 40 с ускоряющими напряжениями 1 и 5 кВ с использованием вторичного электронного детектора. Для различения объектов разного химического состава использовался детектор обратно рассеянных электронов. Изображения во вторичных и обратно рассеянных электронах были получены для одних и тех же точек, чтобы разделить топографический и композиционный контрасты. Дисперсии образцов наносили на кремниевые подложки и сканировали при ускорении электронного пучка напряжением 5 кВ.
Дифрактограммы получены методом порошковой рентгеновской дифракции (ПРД) и измерены с помощью дифрактометра Empyrean (PANalytical), оборудованного 1-D позиционно-чувствительным детектором-X’Celerator для образцов в виде сухих дисперсий, нанесенных на держатели с нулевым фоном кремния. Использовалось Ni-фильтрованное CuKα-излучение. Калибровка угловой шкалы в нижней области была подтверждена с использованием свежего порошка бегената серебра (SigmaAldrich). Была использована стандартная геометрия Брэгга–Брентано (отражение), позволяющая измерять дифракцию вне плоскости.
Спектры комбинационного рассеяния света и фотолюминесценции снимали с помощью системы Senterra (Bruker) при возбуждении светом с длиной волны 532 нм и объективом 50×. Все измерения проводились при мощности возбуждающего лазера менее 2 мВт, чтобы избежать повреждения образца. Спектры снимали в течение 100–500 с в зависимости от образца.
Флуоресцентные фотографии были получены с использованием микроскопа Nikon ECLIPSE Ti. Использовалось десятикратное увеличение, а также для возбуждения излучения лазер с длиной волны λex = 405 нм.
Электронные спектры поглощения в видимой и УФ областях регистрировали на спектрофотометре Jasco V-760 в кварцевых прямоугольных кюветах с длиной оптического пути 10 мм. В качестве источника излучения использовалась фитолампа модель LED-A60-15W/SPSB/E27/CLPLP30WH.
Спектры ЯМР 1H, записывались на спектрометре Bruker Avance III 600 (600.13 МГц). Химические сдвиги (d, м.д.) измеряли при T = 303 K.
Синтез исходных компонентов гибридных систем
Оксид графена был синтезирован в точном соответствии литературной методике [42] и охарактеризован методами СЭМ, АСМ и РФЭС. 2,3,9,10,16,17,23,24-окта[(3,5-бискарбоксилат натрия)фенокси]фталоцианинат цинка(II) (ZnPc16) был синтезирован по описанной в литературе методике [27]. Степень чистоты определена методом ЯМР-спектроскопии: ЯМР 1H (600.13 МГц, D2О): 9.42 (s, 8H, HPc), 8.16 (s, 8H, HP), 7.78 (s, 16H, Ho).
Синтез гибридных материалов ОГ/ZnPc16
Для синтеза гибридных систем на основе ОГ и ZnPc16 был использован метод гидротермального синтеза. Для получения гибридной системы ОГ/ZnPc16 2 мл гидрозоля ОГ (4.8 мг/мл, 9.5 мг) и ZnPc16 (0.5 мг) с соотношением ZnPc16/ОГ 5 мас. %, растворили в 10 мл деионизированной воды и обработали в ультразвуковой бане в течение 30 мин. Затем смесь нагревали при 70°C в шкафу без перемешивания в закрытом сосуде. Полученный материал высушивали в эксикаторе под вакуумом (200 мПа, 23°C).
Для получения гибридных систем ОГ/Zn(OAc)2/ZnPc16 2 мл гидрозоля ОГ (4.8 мг/мл, 9.5 мг) и Zn(OAc)2 ∙ 2H2O (0.9 мг) растворили в 10 мл деионизированной воды, затем обработали в ультразвуковой бане в течение 30 мин. Далее навеску ZnPc16 (0.5 мг) растворяли в полученном растворе ОГ/Zn(OAc)2 с конечным соотношением ZnPc16/ ОГ 5 мас. %. Смешанную дисперсию нагревали при 70°C в шкафу без перемешивания в закрытом сосуде. Полученный материал высушивали двумя разными способами: в эксикаторе под вакуумом (200 мПа, 23°C) и с помощью лиофильной сушилки (2 мПа, –110°C).
Также в качестве контрольного образца сравнения была использована чистая графеновая губка. Для получения контрольного образца 2 мл гидрозоля ОГ (4.8 мг/мл, 9.5 мг) и Zn(OAc)2 ∙ 2H2O (0.9 мг) растворяли в 10 мл деионизированной воды, затем обрабатывали в ультразвуковой бане в течение 30 мин. Полученную смесь нагревали при 70°C в шкафу без перемешивания в закрытом сосуде. Материал высушивали с помощью лиофильной сушилки (2 мПа, –110°C) – ОГ/Zn(OAc)2.
Исследование фотокаталитической активности
Исследование фотокаталитической активности ZnPc16, а также полученных гибридных систем в реакциях окислительной фотодеструкции водных растворов субстратов (Rh6G, ДГН, НФ) проводилось с помощью электронных спектров поглощения. Для этого 1 мг гибридного материала на основе ОГ и ZnPc16 добавляли в 10 мл раствора соответствующего субстрата с концентрацией 1 × 10–5 М. Флакон запечатывали, встряхивали и оставляли на ночь в темноте. Затем записывали электронные спектры поглощения для определения количества адсорбированного субстрата из водного раствора. Для инициирования реакции емкость облучали фитолампой для комнатных растений (430–480 и 620–780 нм, 15 Вт). Для анализа скорости фотодеструкции измеряли спектр поглощения с интервалом в 2 мин. После облучения растворы центрифугировали в течение 1 мин для осаждения взвеси гибридного материала и отбирали для измерения аликвоту объемом 3 мл. Спектральные данные были нормированы относительно максимума.
РЕЗУЛЬТАТЫ И ОБСУЖДЕНИЕ
Исследование фотокаталитической активности ZnPc16
На начальном этапе исследования была изучена фотокаталитическая активность индивидуального ZnPc16 (структурная формула приведена на рис. 1) в реакции окислительной фотодеструкции модельных органических соединений Rh6G, ДГН и НФ в водных растворах (рис. 2). Возбуждение молекул тетрапиррольного соединения проводилось при помощи фитолампы, так как спектр поглощения этого фталоцианината (рис. 3) близок к спектру испускания данного источника в красной области 600–700 нм (рис. 3, вставка), при этом мощность излучения достаточна для фотовозбуждения и не приводит к фотодеградации хромофора. На рис. 4 приведены электронные спектры поглощения растворов (а) Rh6G, (б) ДГН и (в) НФ, С = 1 × 10–5 М, записанные в присутствии ZnPc16, С = 1 × 10–6 М. Как видно из приведенных спектров, интенсивность характеристических полос поглощения субстратов остается неизменной на протяжении всего времени облучения растворов. В то же время наблюдается постепенное уменьшение интенсивности полос поглощения фталоцианинового фотосенсибилизатора ZnPc16 (на вставках рис. 4 приведены спектры поглощения после облучения с увеличением в области 650-700 нм), что свидетельствует о его разрушении в процессе облучения. Таким образом, в условиях, отвечающих условиям гомогенного фотокатализа, ZnPc16 не способен инициировать реакцию фотоокисления субстратов, несмотря на имеющиеся сведения о его способности инициировать генерацию активных кислородсодержащих интермедиатов в водном растворе [27]. Именно вследствие этой способности в сочетании с относительно неустойчивой молекулярной структурой с большим числом заместителей, этот макроциклический сенсибилизатор даже при низких концентрациях подвергается интенсивной автодеструкции под действием света. Увеличение фотохимической устойчивости макроциклической структуры ZnPc16 потенциально может быть обеспечено при ее гибридизации с неорганической наноразмерной матрицей ОГ за счет различных типов нековалентных взаимодействий, что, в свою очередь, может привести к появлению фотокаталитических свойств в отношении выбранных субстратов.
Рис. 4.
Электронные спектры поглощения растворов субстратов (а) Rh6G, (б) ДГН и (в) НФ без и в присутствии ZnPc16 при облучении фитолампой в течение 10 мин, записанные с интервалом в 2 мин. Вставка: увеличенное изображение спектров поглощения в области 650–700 нм (в области поглощения ZnPc16). Стрелками указано снижение интенсивности поглощения ZnPc16.
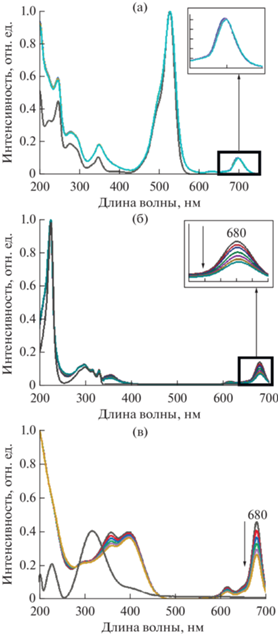
Получение и характеризация ОГ
Для получения гибридных систем на основе ZnPc16 был синтезирован оксид графена по модифицированному методу Хаммерса, согласно описанной ранее методике [42], который позволяет получать однослойные частицы ОГ с необходимой степенью окисления и латеральным размером листов. В результате последовательного окисления графитовой пудры такими сильными окислителями, как персульфат калия, серная кислота, перманганат калия и перекись водорода, происходит разрыв слоистой структуры графита и окисление получившихся отдельных углеродных листов с образованием эпоксидных, гидроксильных и карбоксильных групп с увеличением их локальной концентрации в направлении от центра к краю наночастицы ОГ [42]. После завершения процесса окисления полученный золь подвергается ультразвуковой обработке до достижения необходимого латерального размера частиц. По данным СЭМ для пленки, полученной растеканием разбавленного гидрозоля ОГ на твердой подложке, средний латеральный размер нанолистов ОГ составил около 5 мкм (рис. 5а). С помощью АСМ была подтверждена однослойная структура ОГ. АСМ-изображение и соответствующий профиль поверхности пленки, осажденной на кремниевую подложку из разбавленного гидрозоля, представлены на рис. 5б. Профиль края наночастицы ОГ получен в полуконтактном режиме, высота профиля (то есть, толщина частицы ОГ) составила около 1 нм, что соответствует монослою из окисленного углеродного каркаса. Степень окисления полученного оксида графена определяли с помощью РФЭС, соответствующий спектр представлен на рис. 6. Для оценки соотношения С/О в полученном ОГ был произведен расчет отношения площади под пиком C–C к сумме площадей пиков С–О(ОН) и С=О, которое составило 0.805. Концентрацию золя по углероду (4.8 мг/мл) определяли с учетом данных РФЭС.
Получение и характеризация гибридных материалов ОГ/ZnPc16 и ОГ/Zn(OAc2)/ZnPc16
Стабилизация фотоактивного компонента ZnPc16 на углеродной двумерной матрице ОГ потенциально может достигаться за счет вандерваальсовых, электростатических и ароматических взаимодействий между макроциклом, его заместителями и углеродным каркасом, и функциональными группами ОГ или за счет координационных связей координационного мотива типа “гребное колесо” [43], образующихся при взаимодействии ионов Zn2+ в металлокластере Zn(OAc)2 с карбоксильными группами оксида графена и фталоцианина. Ранее нами уже была успешно реализована стабилизация тетрапиррольных соединений за счет их нековалентной (супрамолекулярной) сборки на поверхности листов ОГ посредством образования такого координационного мотива для формирования и структурирования тонких организованных пленок на основе порфиринов [41]. В то же время аналогичный подход к стабилизации фталоцианинов, таких как ZnPc16, может оказаться связан с трудностями, обусловленными большим размером макроцикла и соответствующими стерическими ограничениями, возникающими при его взаимодействии с ОГ.
Для оценки влияния типа взаимодействий между фталоцианинатом и двумерной углеродной матрицей на свойства и устойчивость ZnPc16 были получены два типа гибридных дисперсных структур. Система состава ОГ/ZnPc16 была получена простым смешиванием ZnPc16 и гидрозоля ОГ и выдержке полученного раствора при 70°С в течение двух дней. Для формирования гибрида ОГ/Zn(OAc)2/ZnPc16) была использована двухстадийная методика, включающая предварительную обработку золя ОГ раствором ацетата цинка, а затем добавлением в раствор сухого ZnPc16. Полученный раствор также выдерживали при 70°C в течение двух дней. Сухие дисперсии обоих типов получали после предварительной промывки и удаления растворителей с помощью вакуумной и/или лиофильной сушки. В качестве контрольной системы сравнения использовали сухую графеновую губку на основе золя ОГ, обработанного ацетатом цинка.
Морфология полученных дисперсий была исследована с помощью метода СЭМ, соответствующие микрофотографии приведены на рис. 7. Согласно полученным данным, гибрид ОГ/ZnPc16 (рис. 7а) обладает морфологией, сходной с морфологией контрольной системы ОГ/Zn(OAc)2 (рис. 7г). Такая морфология характерна для твердодисперсных систем, состоящих из агрегировавших нанолистов ОГ с неровными краями [44]. Микроскопический анализ сухих гибридных дисперсий, полученных с использованием металлокластера, выявил зависимость морфологии таких систем от способа удаления растворителя. Морфология поверхности гибрида ОГ/Zn(OAc)2/ZnPc16, полученного с помощью вакуумной сушки, обладает большей неоднородностью по сравнению с композитами более простого состава (рис. 7б). На микрофотографиях можно различить отдельные светлые частицы выделившейся фазы, наиболее вероятно состоящие из соли металла, что указывает на возможное фазовое разделение компонентов системы в процессе сушки материала. В то же время аналогичный гибрид ОГ/Zn(OAc)2/ZnPc16, полученный с помощью лиофильной сушки, состоит из агрегатов протяженных частиц с игольчатой морфологией, близких по размеру (30 ± 10 мкм). Поверхность частиц однородна и не содержит отдельных включений (рис. 7в). Очевидно, быстрое удаление растворителя предотвращает разрушение структуры материала, сформированной в процессе получения, и, как следствие, ингибирует фазовый распад и агрегацию отдельных компонентов системы.
Рис. 7.
Микрофотографии СЭМ гибридных систем: (а) ОГ/ZnPc16; (б) ОГ/Zn(OAc)2/ZnPc16, высушенного под вакуумом и (в) высушенного с помощью лиофильной сушки; (г) контроль – ОГ/Zn(OAc)2.
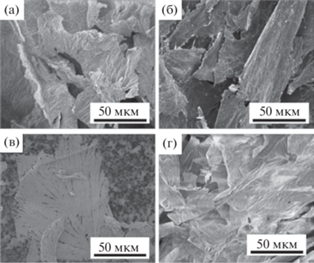
Методом рентгеновской порошковой дифракции была исследована структура полученных гибридных дисперсий (рис. 8). Ранее нами было показано, что предварительная обработка листов ОГ ацетатом цинка способствует формированию упорядоченных кластеров и пленок из полиароматических соединений, таких как карбоксил-замещенные производные бис-перилена или порфирината цинка, за счет формирования координационных связей с затравочным слоем металлокластера [39–41]. В случае же гибридизации ОГ и карбоксил-замещенного фталоцианината ZnPc16, из полученных результатов следует, что такой способ интеграции компонентов не приводит к формированию упорядоченной структуры, при этом следует иметь в виду, что индивидуальный ZnPc16 в силу молекулярной структуры не образует кристаллической фазы. Дифракционная картина для ОГ/Zn(OAc)2/ZnPc16, высушенного под вакуумом (рис. 8, кривая 1), содержит пики отдельных компонентов, аморфизованного фталоцианината в области малых углов и кристаллического Zn(OAc)2 (пики в области 12, 19 и 24 град), что согласуется с данными микроскопического исследования и подтверждает сделанное предположение о фазовом распаде такой дисперсии. (Для последующего анализа функциональных свойств использовалась только однородная гибридная система ОГ/Zn(OAc)2/ZnPc16, полученная методом лиофильной сушки).
Рис. 8.
Дифрактограммы: ОГ/Zn(OAc)2/ZnPc16 высушенного под вакуумом (1) и с помощью лиофильной сушки (2), ОГ/ZnPc16 (3), ОГ/Zn(OAc)2 (4).
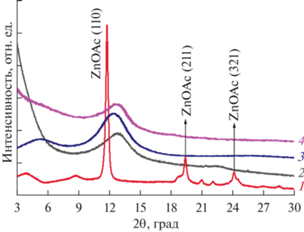
Картины рентгеновской дифракции для гибрида ОГ/Zn(OAc)2/ZnPc16, полученного с помощью лиофильной сушки (рис. 8, кривая 2), и ОГ/ZnPc16 совпадают (рис. 8, кривая 3) и содержат единственный пик в области 2θ ~ 13°, который является характеристическим для чистого ОГ (рис. 8, кривая 4). По-видимому, стерический фактор является определяющим для закрепления ZnPc16 на поверхности листов ОГ, а молекулярное строение ZnPc16 препятствует межмолекулярной самоорганизации фотосенсибилизатора в упорядоченные агрегаты на двумерной матрице независимо от типа взаимодействий между макроциклом и ОГ.
Структура полученных образцов изучалась также с помощью спектроскопии комбинационного рассеяния. Спектр ОГ/ZnPc16 содержит характеристические D и G пики ОГ (рис. 9, кривые 1 и 3) [45], а также слабо выраженный характеристический пик в области 1515 см–1, подтверждающий наличие фталоцианина в материале. Спектр гибрида ОГ/Zn(OAc)2/ZnPc16 включает в себя как пик ОГ в области 1600 см–1, так и выраженные пики фталоцианината с небольшим батохромным сдвигом (рис. 9, кривые 2 и 4): 1125 против 1128, 1328 против 1330, 1456 против 1460 см–1. Наблюдаемые различия в спектрах КР очевидно обусловлены различием в строении полученных материалов. Несмотря на равное относительно низкое содержание ZnPc16 в полученных материалах, только в одном удается получить хорошо разрешенные пики макроцикла. Интересно, что сходная зависимость эффекта усиления КР от структуры полиароматического соединения ранее наблюдалась нами для производных перилена различного строения на поверхности монослоев ОГ на твердых подложках. Усиление характеристических линий не достигалось для молекулы, содержащей боковые заместители, препятствующие стекингу периленового ядра и углеродного каркаса [46]. Тем не менее, поскольку выделение фталоцианина в отдельную микронизированную флуоресцирующую фазу в гибридах невозможно определить с помощью рентгеновских исследований, вопрос о причине выявленных спектральных различий требует отдельного изучения с привлечением других оптических методов, в том числе, времяразрешенной флуоресцентной спектроскопии.
Оптические свойства полученных сухих дисперсий были изучены с помощью флуоресцентной микроскопии. На рис. 10 приведены микрофотографии, полученные с увеличением 10x для гибридных систем (а) ОГ/ZnPc16 и (б) ОГ/Zn(OAc)2/ZnPc16. Возбуждение флуоресценции проводили при облучении сухих образцов лазером с λex = 405 нм, соответствующей полосе поглощения фталоцианина в коротковолновой области, для устранения побочной флуоресценции и предотвращения фотоиндуцированной деградации хромофора. Из приведенных парных оптических и флуоресцентных изображений микрочастиц видно, что оба типа дисперсий обладают выраженной флуоресценцией. Этот результат указывает на успешную интеграцию ZnPc16 с неорганической двумерной матрицей ОГ с сохранением фотохимических свойств макроциклического сенсибилизатора в обоих случаях. В то же время в гибриде ОГ/Zn(OAc)2/ZnPc16 флуоресценция несколько менее выражена, что согласуется с данными КР о лучшем разрешении спектра макроцикла в этом материале, так как флуоресценция макроцикла может препятствовать проявлению его спектральных линий КР. Таким образом, результаты характеризации полученных гибридных материалов позволяют предполагать, что благодаря интеграции ОГ и фотосенсибилизатора без его разрушения, облучение этих систем видимым светом может приводить к генерации активных интермедиатов в водных средах и катализу фотоокислительной деструкции органических субстратов.
Исследование сорбционных и фотокаталитических свойств гибридных дисперсий ОГ/ZnPc16 и ОГ/Zn(OAc)2/ZnPc16
Исследование сорбционной способности гибридных материалов ОГ/ZnPc16 и ОГ/Zn(OAc)2/ZnPc16 в отношении ряда органических соединений проводилось спектрофотометрическим методом. На рис. 11 приведены спектры поглощения растворов модельных субстратов-поллютантов (а) Rh6G, (б) ДГН и (в) НФ до и после установления равновесного состояния в присутствии гибридных материалов. Уменьшение интенсивности полос поглощения субстратов в растворах, выдержанных в присутствии гибридных дисперсий, свидетельствует о способности полученных систем к адсорбции органических соединений из растворов. В табл. 1 приведены относительные величины адсорбции, рассчитанные как интегральное отношение площадей соответствующих спектров, записанных в начальный момент времени и после установления равновесия. Согласно приведенным данным, контрольный образец чистого ОГ не проявляет выраженного сродства по отношению к какому-либо из выбранных субстратов и обладает низкой адсорбционной способностью относительно всех исследованных органических молекул (интегральное снижение интенсивности поглощения субстратов не превышает 15%). В то же время оба типа гибридных систем ОГ/ZnPc16 и ОГ/Zn(OAc)2/ZnPc16 проявляют выраженные адсорбционные свойства по отношению к молекулам Rh6G и НФ по сравнению с контрольной системой на основе ОГ, не содержащей фталоцианината, при этом относительное снижение интенсивности для каждого из этих субстратов в присутствии для этих систем приблизительно одинаково. Интересно, что в отношении ДГН гибридные системы не проявляют высокой адсорбционной активности, которая в обоих случаях оказалась сравнима с измеренной для чистого ОГ. Можно предположить, что связывание определенного субстрата гибридными системами определяется в первую очередь именно рецепторными свойствами фталоцианинового лиганда ZnPc16. По-видимому, это соединение, которое потенциально способно координировать различные функциональные группы на металлоцентре, образовывать водородные связи и ионные пары с помощью своих карбоксильных заместителей, а также вступать в ароматические взаимодействия с органическими молекулами, обладает разным сродством по отношению к выбранным субстратам. Поскольку наименьшую адсорбционную активность гибридные системы проявляют по отношению к ДГН, вероятно, ароматические стекинговые взаимодействия с субстратом являются наименее выгодными для ZnPc16, адсорбированного на поверхности углеродной матрицы, также следует учитывать и способность субстратов к ионизации в условиях эксперимента, однако этот аспект также требует дальнейшего изучения.
Рис. 11.
Электронные спектры поглощения растворов (а) Rh6G, (б) ДГН и (в) НФ, после адсорбции и достижения равновесия в присутствии ОГ/Zn(OAc)2/ZnPc16.
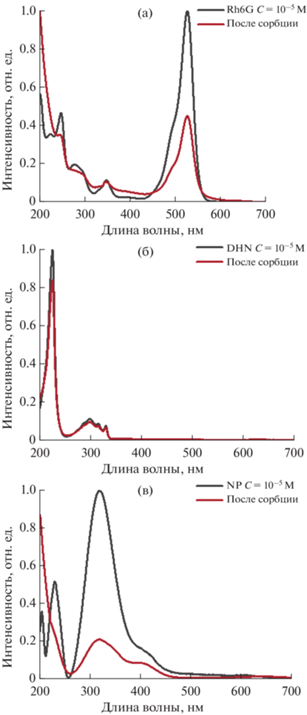
Таблица 1.
Адсорбция субстратов из водных растворов в присутствии гибридных материалов и контрольного ОГ в относительных процентах.
Субстрат | Адсорбция, отн. % | ||
---|---|---|---|
ОГ/ZnPc16 | ОГ/Zn(OAc)2/ZnPc16 | ОГ | |
Rh6G | 58 | 55 | 10 |
ДГН | 14 | 16 | 15 |
НФ | 62 | 65 | 14 |
После установления равновесного состояния была исследована фотокаталитическая активность гибридных материалов ОГ/ZnPc16 и ОГ/Zn(OAc)2/ZnPc16 при облучении растворов субстратов фитолампой в течение 10 мин. На рис. 12 и 13 приведены спектры поглощения (а) Rh6G, (б) ДГН и (в) НФ в присутствии ОГ/ZnPc16 и ОГ/Zn(OAc)2/ZnPc16 соответственно. Из представленных данных видно, что, несмотря на выявленные флуоресцентные и адсорбционные свойства, ОГ/ZnPc16 остается полностью инертным и не вызывает снижения интенсивности характеристических полос Rh6G и ДГН, то есть не проявляет фотокаталитической активности в их отношении (рис. 12). Некоторая активность была выявлена для этого гибрида в отношении НФ, однако степень конверсии (т.е., окислительной деградации вещества-поллютанта в растворе) составила всего 6% за 10-минутный интервал, что соответствует скорости реакции 5.4 × 10–3 мин–1.
Рис. 12.
Электронные спектры поглощения и соответствующие кинетические зависимости фотодеградации для растворов (а) Rh6G, (б) ДГН и (в) НФ в присутствии ОГ/ZnPc16 при облучении фитолампой в течение 10 минут, снятые с интервалом 2 мин. Вставка: увеличенное изображение спектров поглощения НФ в области 280–340 нм.
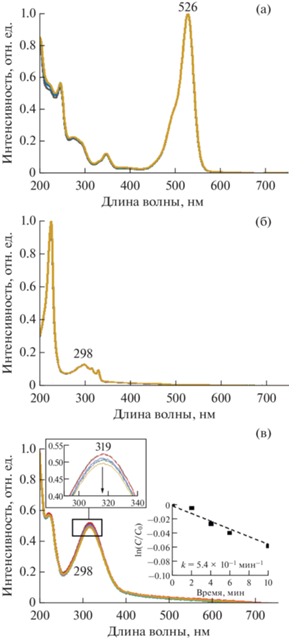
Рис. 13.
Электронные спектры поглощения и соответствующие кинетические зависимости фотодеградации (а) Rh6G, (б) ДГН и (в) НФ в присутствии ОГ/Zn(OAc)2/ZnPc16, при облучении фитолампой в течение 10 мин, снятые с интервалом 2 мин. Вставка: увеличенное изображение спектров поглощения ДГН в области 260–340 нм.
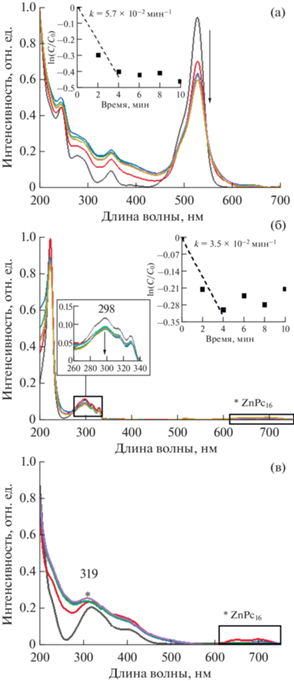
По-видимому, взаимодействия макроциклического фотосенсибилизатора и ОГ в гибриде такого типа приводят к передаче энергии фотовозбуждения преимущественно на углеродный каркас, а не на молекулы воды и растворенный в ней кислород, с последующей диссипацией.
В отличие от гибрида ОГ/ZnPc16, способность гибрида ОГ/Zn(OAc)2/ZnPc16 вызывать фотоиндуцированную деградацию спектров органических молекул достаточно высока, но при этом не демонстрирует прямой корреляции с его адсорбционными свойствами – уменьшение интенсивности характеристических полос наблюдается для растворов Rh6G и ДГН и не обнаружено для НФ (рис. 13). Скорость фотодеградации для Rh6G в присутствии этого гибрида составила 5.7 × 10–2 мин–1, для ДГН – 3.5 × 10–2 мин–1. В то же время максимальная конверсия вещества (37 и 26% соответственно) достигалась в обоих случаях в течение первых пяти минут облучения растворов, а дальнейшее облучение не приводило к существенному снижению интенсивности характеристических полос субстратов, при этом постепенно нарастал разброс измеряемых величин. Кроме того, при измерении в растворе НФ в спектрах раствора начинают появляться характеристические полосы ZnPc16 с нарастающей интенсивностью. Совокупность полученных данных указывает на то, что взаимодействия между ОГ и ZnPc16 в гибриде, сформированном с помощью металлокластера, хотя и позволяют достичь фотокаталитической активности гибридной системы, но не обеспечивают ее необходимой структурной устойчивости в водной среде.
ЗАКЛЮЧЕНИЕ
Полученные в работе результаты указывают на возможность интеграции таких активных фотосенсибилизаторов и генераторов синглетного кислорода, как карбоксил-замещенные фталоцианинаты, с оксидом графена для получения гибридных структур различного типа за счет нековалентных взаимодействий, как непосредственных между неорганическим и органическим компонентами, так и реализуемых посредством формирования координационных связей между металлокластером ацетата цинка(II), неорганической матрицей и макроциклическим лигандом. Гибридизация водорастворимого фталоцианината цинка(II) с оксидом графена позволяет предотвратить деградацию структуры неустойчивого к воздействию света соединения и его флуоресцентных свойств. Гибриды обоих типов проявляют сходное избирательное сродство по отношению к органическим субстратам, что наиболее вероятно обусловлено рецепторными свойствами металлокомплекса. В то же время только гибридизация с использованием связующего металлокластера позволяет инициировать появление фотокаталитических свойств, а непосредственные взаимодействия между углеродным каркасом и фталоцианинатом, по-видимому, приводят к диссипации энергии фотовозбуждения макроцикла на углеродной матрице. Полученные данные о фотокаталитической активности гибридных систем, сформированных нековалентной сборкой за счет координационных связей, открывают принципиальную возможность для дальнейшего развития этого подхода к дизайну новых гибридных материалов на основе высокоактивных фотосенсибилизаторов. Тем не менее относительно низкая структурная устойчивость таких систем требует особого внимания к подбору фталоцианинатов с оптимальными структурными характеристиками, прежде всего числом и типом функциональных заместителей, для решения этой проблемы.
Список литературы
Gyamfi B.A., Onifade S.T., Nwani C., Bekun F.V. Accounting for the combined impacts of natural resources rent, income level, and energy consumption on environmental quality of G7 economies: A panel quantile regression approach // Environmental Science and Pollution Research. 2022. V. 29. № 2. P. 2806–2818. https://doi.org/10.1007/s11356-021-15756-8
Ebhota W.S., Jen T.C. Fossil fuels environmental challenges and the role of solar photovoltaic technology advances in fast tracking hybrid renewable energy system // International Journal of Precision Engineering and Manufacturing – Green Technology. 2020. V. 7. № 1. P. 97–117. https://doi.org/10.1007/s40684-019-00101-9
Mazzeo A., Santalla S., Gaviglio C., Doctorovich F., Pellegrino J. Recent progress in homogeneous light-driven hydrogen evolution using first-row transition metal catalysts // Inorganica Chimica Acta. 2020. V. 517. P. 119950. https://doi.org/10.1016/j.ica.2020.119950
Whittemore T.J., Xue C., Huang J., Gallucci J.C., Turro C. Single-chromophore single-molecule photocatalyst for the production of dihydrogen using low-energy light // Nature Chemistry. 2020. V. 12. № 2. P. 180–185. https://doi.org/10.1038/s41557-019-0397-4
Zhang Y., Ren K., Wang L.L., Wang L.L., Fan Z. Porphyrin-based heterogeneous photocatalysts for solar energy conversion // Chinese Chemical Letters. 2022. V. 33. № 1. P. 33–60. https://doi.org/10.1016/j.cclet.2021.06.013
Liu M.L., Guo J.L., Japip S. et al. One-step enhancement of solvent transport, stability and photocatalytic properties of graphene oxide/polyimide membranes with multifunctional cross-linkers // Journal of Materials Chemistry A. 2019. V. 7. № 7. P. 3170–3178. https://doi.org/10.1039/C8TA11372F
Liu X., Chen Q., Lv L., Feng X., Meng X. Preparation of transparent PVA/TiO2 nanocomposite films with enhanced visible-light photocatalytic activity // Catalysis Communications. 2015. V. 58. P. 30–33. https://doi.org/10.1016/j.catcom.2014.08.032
Das P., Chakraborty K., Chakrabarty S., Ghosh S., Pal T. Reduced graphene oxide – zinc phthalocyanine composites as fascinating material for optoelectronic and photocatalytic applications // ChemistrySelect. 2017. V. 2. № 11. P. 3297–3305. https://doi.org/10.1002/slct.201700384
Zhang Z., Wang J., Liu D. et al. Highly efficient organic photocatalyst with full visible light spectrum through π–π stacking of TCNQ-PTCDI // ACS Applied Materials and Interfaces. 2016. V. 8. № 44. P. 30225–30231. https://doi.org/10.1021/acsami.6b10186
Mak C.H., Han X., Du M. et al. Heterogenization of homogeneous photocatalysts utilizing synthetic and natural support materials // Journal of Materials Chemistry A. 2021. V. 9. № 8. P. 4454–4504. https://doi.org/10.1039/D0TA08334H
Anaya-Rodríguez F., Durán-Álvarez J.C., Drisya K.T., Zanella R. The challenges of integrating the principles of green chemistry and green engineering to heterogeneous photocatalysis to treat water and produce green H2 // Catalysts. 2023. V. 13. № 1. P. 154. https://doi.org/10.3390/catal13010154
Xu C., Ravi Anusuyadevi P., Aymonier C., Luque R., Marre S. Nanostructured materials for photocatalysis // Chemical Society Reviews. 2019. V. 48. № 14. P. 3868–3902. https://doi.org/10.1039/C9CS00102F
Liu Y., Tian J., Wei L. et al. Modified g-C3N4/TiO2/CdS ternary heterojunction nanocomposite as highly visible light active photocatalyst originated from CdS as the electron source of TiO2 to accelerate Z-type heterojunction // Separation and Purification Technology. 2021. V. 257. P. 117976. https://doi.org/10.1016/j.seppur.2020.117976
Zhang X.Y., Li H.P., Cui X.L., Lin Y. Graphene/TiO2 nanocomposites: Synthesis, characterization and application in hydrogen evolution from water photocatalytic splitting // Journal of Materials Chemistry. 2010. V. 20. № 14. P. 2801–2806. https://doi.org/10.1039/B917240H
Nemiwal M., Zhang T.C., Kumar D. Recent progress in g-C3N4, TiO2 and ZnO based photocatalysts for dye degradation: Strategies to improve photocatalytic activity // Science of the Total Environment. 2021. V. 767. P. 144896. https://doi.org/10.1016/j.scitotenv.2020.144896
Hsu H.C., Shown I., Wei H.Y. et al. Graphene oxide as a promising photocatalyst for CO2 to methanol conversion // Nanoscale. 2013. V. 5. № 1. P. 262–268. https://doi.org/10.1039/C2NR31718D
Zhao Y.S., Yao J. Organic one-dimensional nanostructures: Construction and optoelectronic properties // In: Zhai T., Yao J. (Eds). One-Dimensional Nanostructures: Principles and Applications. John Wiley & Sons, Hoboken, 2013. P. 381–395. https://doi.org/10.1002/9781118310342.ch17
Chen Y.Z., Li W.H., Li L., Wang L.N. Progress in organic photocatalysts // Rare Metals. 2018. V. 37. № 1. P. 1–12. https://doi.org/10.1007/s12598-017-0953-2
Kosco J., Moruzzi F., Willner B., McCulloch I. Photocatalysts based on organic semiconductors with tunable energy levels for solar fuel applications // Advanced Energy Materials. 2020. V. 10. № 39. P. 2001935. https://doi.org/10.1002/aenm.202001935
Díaz U., Brunel D., Corma A. Catalysis using multifunctional organosiliceous hybrid materials // Chemical Society Reviews. 2013. V. 42. № 9. P. 4083–4097. https://doi.org/10.1039/C2CS35385G
Arslanov V.V., Kalinina M.A., Ermakova E.V., Raitman O.A., Gorbunova Y.G. et al. Hybrid materials based on graphene derivatives and porphyrin metal-organic frameworks // Russian Chemical Reviews. 2019. V. 88. № 8. P. 775–799. https://doi.org/10.1070/RCR4878
Zhao G., Pang H., Liu G. et al. Co-porphyrin/carbon nitride hybrids for improved photocatalytic CO2 reduction under visible light // Applied Catalysis B: Environmental. 2017. V. 200. P. 141–149. https://doi.org/10.1016/j.apcatb.2016.06.074
Нугманова А.Г., Калинина М.А. Cупрамолекулярная самосборка гибридных коллоидных систем // Коллоидный журнал. 2022. Т. 84. № 5. С. 669–692. https://doi.org/10.31857/S0023291222600213
Gacka E., Burdzinski G., Marciniak B., Kubas A., Lewandowska-Andralojc A. Interaction of light with a non-covalent zinc porphyrin–graphene oxide nanohybrid // Physical Chemistry Chemical Physics. 2020. V. 22. № 24. P. 13456–13466. https://doi.org/10.1039/D0CP02545C
Sorokin A.B. Phthalocyanine metal complexes in catalysis // Chemical Reviews. 2013. V. 113. № 10. P. 8152–8191. https://doi.org/10.1021/cr4000072
Jin L., Lv S., Miao Y., Liu D., Song F. Recent development of porous porphyrin-based nanomaterials for photocatalysis // ChemCatChem. 2021. V. 13. № 1. P. 140–152. https://doi.org/10.1002/cctc.202001179
Liu W., Jensen T.J., Fronczek F.R. et al. Synthesis and cellular studies of nonaggregated water-soluble phthalocyanines // Journal of Medicinal Chemistry. 2005. V. 48. № 4. P. 1033–1041. https://doi.org/10.1021/jm049375b
Gregory P. Industrial applications of phthalocyanines // Journal of Porphyrins and Phthalocyanines. 2000. V. 4. № 4. P. 432–437. https://doi.org/10.1002/(SICI)1099-1409(200006/07)4:4<432::AID-JPP254>3.0.CO;2-N
Nikoloudakis E., López-Duarte I., Charalambidis G., Ladomenou K., Ince M., Coutsolelos A.G. Porphyrins and phthalocyanines as biomimetic tools for photocatalytic H2 production and CO2 reduction // Chemical Society Reviews. 2022. V. 51. № 16. P. 6965–7045. https://doi.org/10.1039/D2CS00183G
Liu Y., Zuo P., Wang F. et al. Covalent immobilization of phthalocyanine on graphene oxide for the degradation of phenol // Journal of the Taiwan Institute of Chemical Engineers. 2019. V. 104. P. 187–200. https://doi.org/10.1016/j.jtice.2019.09.007
Qian J., Liu Y., Zheng W., Zhou B., Dong X. Covalent modification of iron phthalocyanine into skeleton of graphitic carbon nitride and its visible-light-driven photocatalytic reduction of nitroaromatic compounds // Catalysts. 2022. V. 12. № 7. P. 752. https://doi.org/10.3390/catal12070752
Jiang B.P., Hu L.F., Wang D.J. et al. Graphene loading water-soluble phthalocyanine for dual-modality photothermal/photodynamic therapy via a one-step method // Journal of Materials Chemistry B. 2014. V. 2. № 41. P. 7141–7148. https://doi.org/10.1039/C4TB01038H
Kumar P., Kumar A., Sreedhar B. et al. Cobalt phthalocyanine immobilized on graphene oxide: An efficient visible-active catalyst for the photoreduction of carbon dioxide // Chemistry – A European Journal. 2014. V. 20. № 20. P. 6154–6161. https://doi.org/10.1002/chem.201304189
Dyrda G., Kocot K., Poliwoda A. et al. Hybrid TiO2 @ phthalocyanine catalysts in photooxidation of 4-nitrophenol: Effect of the matrix and sensitizer type // Journal of Photochemistry and Photobiology A: Chemistry. 2020. V. 387. P. 112124. https://doi.org/10.1016/j.jphotochem.2019.112124
Prasad C., Liu Q., Tang H. et al. An overview of graphene oxide supported semiconductors based photocatalysts: Properties, synthesis and photocatalytic applications // Journal of Molecular Liquids. 2020. V. 297. P. 111826. https://doi.org/10.1016/j.molliq.2019.111826
Lu K.Q., Li Y.H., Tang Z.R., Xu Y.J. Roles of graphene oxide in heterogeneous photocatalysis // ACS Materials Au. 2021. V. 1. № 1. P. 37–54. https://doi.org/10.1021/acsmaterialsau.1c00022
Motevalli B., Fox B.L., Barnard A.S. Charge-dependent Fermi level of graphene oxide nanoflakes from machine learning // Computational Materials Science. 2022. V. 211. P. 111526. https://doi.org/10.1016/j.commatsci.2022.111526
Hu X., Mu L., Wen J., Zhou Q. Covalently synthesized graphene oxide-aptamer nanosheets for efficient visible-light photocatalysis of nucleic acids and proteins of viruses // Carbon. 2012. V. 50. № 8. P. 2772–2781. https://doi.org/10.1016/j.carbon.2012.02.038
Nugmanova A.G., Kalinina M.A. Self-assembly of metal-organic frameworks in pickering emulsions stabilized with graphene oxide // Colloid Journal. 2021. V. 83. № 5. P. 614–626. https://doi.org/10.1134/S1061933X21050094
Meshkov I.N., Zvyagina A.I., Shiryaev A.A. et al. Understanding self-assembly of porphyrin-based SURMOFs: How layered minerals can be useful // Langmuir. 2018. V. 34. № 18. P. 5184–5192. https://doi.org/10.1021/acs.langmuir.7b04384
Nugmanova A.G., Safonova E.A., Baranchikov A.E. et al. Interfacial self-assembly of porphyrin-based SU-RMOF/graphene oxide hybrids with tunable pore size: An approach toward size-selective ambivalent heterogeneous photocatalysts // Applied Surface Science. 2022. V. 579. P. 152080. https://doi.org/10.1016/j.apsusc.2021.152080
Sladkevich S., Gun J., Prikhodchenko P.V. et al. Peroxide induced tin oxide coating of graphene oxide at room temperature and its application for lithium ion batteries // Nanotechnology. 2012. V. 23. № 48. P. 485601. https://doi.org/10.1088/0957-4484/23/48/485601
Vagin S.I., Ott A.K., Rieger B. Paddle-wheel zinc carboxylate clusters as building units for metal-organic frameworks // Chemie Ingenieur Technik. 2007. V. 79. № 6. P. 767–780. https://doi.org/10.1002/cite.200700062
Jaafar E., Kashif M., Sahari S.K. et al. Study on morphological, optical and electrical properties of graphene oxide (GO) and reduced graphene oxide (rGO) // Materials Science Forum. 2018. V. 917. P. 112–116. https://doi.org/10.4028/www.scientific.net/MSF.917.112
Gusarova E.A., Zvyagina A.I., Aleksandrov A.E. et al. Interfacial self-assembly of ultrathin polydiacetylene/graphene oxide nanocomposites: A new method for synergetic enhancement of surface charge transfer without doping // Colloid and Interface Science Communications. 2022. V. 46. P. 100575. https://doi.org/10.1016/j.colcom.2021.100575
Zvyagina A.I., Gusarova E.A., Averin A.A. et al. Structural effect of perylene derivatives on their interaction with reduced graphene oxide monolayers // Russian Journal of Inorganic Chemistry. 2021. V. 66. P. 273–280. https://doi.org/10.1134/S0036023621020224
Дополнительные материалы отсутствуют.
Инструменты
Коллоидный журнал