Коллоидный журнал, 2023, T. 85, № 5, стр. 641-654
Влияние биологической загрязненности медных поверхностей с экстремальным смачиванием на их антибактериальные свойства
Ф. Ш. Омран 1, *, В. В. Каминский 2, К. А. Емельяненко 1, А. М. Емельяненко 1, Л. Б. Бойнович 1
1 Институт физической химии и электрохимии им. А.Н. Фрумкина РАН
119071 Москва, Ленинский просп. 31, корп. 4, Россия
2 Федеральное государственное бюджетное учреждение “Российский научный центр рентгенорадиологии” Министерства здравоохранения Российской Федерации
117997 Москва, ул. Профсоюзная, д. 86, Россия
* E-mail: duckyfriedrich@gmail.com
Поступила в редакцию 27.06.2023
После доработки 19.07.2023
Принята к публикации 28.07.2023
- EDN: ECUJVB
- DOI: 10.31857/S0023291223600499
Аннотация
Бактериальные инфекции, связанные с оказанием медицинской помощи (ИСМП), являются одной из острых проблем современного здравоохранения. Одним из перспективных направлений решения этой проблемы является разработка материалов, которые либо обладают бактерицидным действием по отношению к возбудителям ИСМП, либо препятствуют передаче попадающих на их поверхность бактерий при контактах пациентов и персонала с такими поверхностями. В данной работе исследована антибактериальная эффективность применения медных контактных поверхностей с различной смачиваемостью. Особое внимание уделено изучению влияния на эту эффективность загрязнения поверхностей как потожировыми выделениями при контактах с людьми, так и поддерживающими жизнедеятельность бактерий веществами на примере пептонного раствора. В связи с дороговизной меди, исследована также возможность замены объемного медного материала на менее дорогой с напыленным медным покрытием. Результаты тестирования показали, что бактерицидная эффективность по отношению к штамму Staphylococcus aureus как контрольных медных и супергидрофильных медных пластин, так и напыленных медных пленок оказывается близкой к 100% и практически не изменяется после загрязнения пептонным раствором или потожировыми выделениями. Супергидрофобные медные поверхности обладают меньшей бактерицидной эффективностью, но благодаря эффекту несмачиваемости и низкой адгезии клеток к такой поверхности, дольше остаются неконтаминированными и тем самым также способствуют снижению передачи инфекций через изготовленные из них поверхности касания.
ВВЕДЕНИЕ
Одной из главных проблем в сфере здравоохранения на сегодняшний день является борьба с распространением инфекций, которые связаны с медицинской помощью (ИСМП) и называются “нозокомиальными”, “внутрибольничными” или “госпитальными”. Это означает, что инфекция развивается у пациента в процессе лечения в больнице или другом медицинском учреждении и отсутствовала на момент поступления. Кроме того, такие инфекции могут поражать и персонал медицинских учреждений [1]. Эта проблема является наиболее частым неблагоприятным последствием медицинской помощи, и ни одно учреждение или страна не может утверждать, что эта проблема уже решена [2, 3]. Данные, полученные в ряде стран, свидетельствуют о том, что ежегодно сотни миллионов пациентов во всем мире страдают от внутрибольничных инфекций [4].
Наиболее распространенные бактерии, вызывающие внутрибольничные инфекции, включают Staphylococcus aureus (S. aureus), в том числе устойчивый к антибиотикам MRSA – метициллинрезистентный золотистый стафилококк (methicillin-resistant S. aureus), Escherichia coli, Enterococcus spp. и Candida spp. [5]. Опасность таких инфекций заключается в высокой резистентности к антибиотикам и антибактериальным средствам, которые широко используют в медицинских учреждениях, что значительно затрудняет их лечение. По этой причине поиск альтернативных способов борьбы с внутрибольничными инфекциями является крайне перспективным и важным направлением исследований.
Одним из таких направлений является внедрение в практику использования материалов, обладающих антимикробными свойствами, например, некоторых металлов (серебро, медь, цинк, висмут [6, 7]) и их сплавов.
Антимикробные свойства меди и ее сплавов известны человечеству уже очень продолжительное время. В современной научной медицине выявлены противовоспалительные [8], иммуномодулирующие [9], противоопухолевые, антимикробные свойства медьсодержащих веществ [10–12]. Медь играет важную роль в работе ряда ферментов – тирозиназы, цитохромоксидазы, а также стимулирует кроветворную функцию костного мозга.
Применение меди в лечебных заведениях в качестве покрытия контактных поверхностей, таких как перила, ручки, двери, столешницы и многое другое, может помочь в борьбе с внутрибольничными инфекциями. Такие исследования уже проводились и показали положительные результаты [13–15].
Однако, несмотря на свои полезные антимикробные свойства, медь оказывает токсическое действие на человека: угнетает центральную нервную систему, вплоть до развития болезни Альцгеймера, способствует инактивации ферментов и угнетению дыхания [16–18].
В настоящее время активно идет обсуждение возможности применения антибактериальных свойств наночастиц и металлических поверхностей с иерархической шероховатостью, сформированной жестко закрепленными наночастицами [19], которые появляются при формировании иерархической шероховатости. В зоне воздействия лазерного луча происходит нагрев поверхности до высокой температуры, плавление, возгонка и взрывная абляция материала. При этом частицы материала удаляются с поверхности и впоследствии осаждаются из лазерного факела на поверхность вокруг зоны воздействия в виде микро- и наночастиц [20]. Иерархическая шероховатость позволяет создать материалы с экстремальной смачиваемостью – супергидрофильные или супергидрофобные. Механизмы антимикробного действия таких материалов находятся в стадии активного изучения [19, 21–23]. Благодаря высокой смачиваемости супергидрофильных поверхностей, бактериальная дисперсия может свободно растекаться, распределяя бактериальные клетки по большой площади. Часть бактерий осаждается на шероховатую поверхность, что приводит к их гибели за счет перфорирования и деформации мембраны элементами нанотекстуры и потери внутриклеточной жидкости. Дополнительно, большая площадь контакта позволяет цитотоксичным ионам меди эффективнее переходить в жидкость и при контакте с бактериальными клетками вызывать окислительный стресс последних [23].
Супергидрофобные поверхности имеют иные механизмы антибактериального действия. На ранних стадиях контакта с бактериальной средой водоотталкивание и гетерогенный режим смачивания приводят к значительному уменьшению площади контакта между подложкой и бактериальной дисперсией и, таким образом, к уменьшению площади, на которой может происходить адгезия бактерий. Второй механизм связан с уменьшением адгезии между бактериальной клеткой и супергидрофобной поверхностью [24]. Это ингибирует закрепление клеток на поверхности материала и дальнейшее формирование биопленок. При длительном контакте супергидрофобной поверхности с бактериальной средой развиваются коррозионные процессы, которые способствуют деградации супергидрофобного состояния и включению механизмов, аналогичных упомянутым для супергидрофильных поверхностей.
Объединение антибактериальных свойств металлов и особенностей поверхностей с иерархической шероховатостью является многообещающим направлением исследований по борьбе с внутрибольничными инфекциями. Ранее в работах нашей лаборатории уже изучалось действие супергидрофобных и супергидрофильных медных поверхностей на некоторые штаммы бактерий [20, 21, 23, 25–27]. Лучшее токсическое действие показали медные супергидрофильные поверхности, что объяснялось более эффективным контактом каждой бактериальной клетки с поверхностью благодаря полному смачиванию поверхности супергидрофильной меди. Распределяясь по шероховатой текстуре, бактериальные клетки подвергались как физическому действию наночастиц меди на клеточные мембраны, так и активному воздействию ионов меди, негативно влияющих на жизнедеятельность бактериальных клеток.
Супергидрофобные поверхности из-за высокого угла смачивания ограничивали площадь непосредственного контакта бактериальной суспензии с поверхностью. Токсическое действие начинало проявляться только спустя некоторое время, когда начинали развиваться процессы коррозии, и ионы меди попадали в бактериальную дисперсию. Однако основные механизмы антибактериального действия супергидрофобных поверхностей при кратковременном контакте с бактериально загрязненными средами заключаются в минимизации площади контакта бактериальных клеток с такой поверхностью и в снижении первичной адгезии клеток к поверхности за счет электростатических и стерических взаимодействий [27]. Оба упомянутых механизма способствуют более длительному сохранению бактериальной чистоты супергидрофобной поверхности и препятствуют контактной передаче инфекций.
Еще одним плюсом в использовании медных поверхностей с экстремальным смачиванием вместо свободных наночастиц меди является то, что наночастицы на обработанных лазером шероховатых поверхностях жестко сцеплены с ними и не могут попасть в организм человека, вызывая негативные последствия, описанные выше.
Однако медь является дорогим материалом. Поэтому применение цельнометаллических изделий для оборудования в больницах будет сопряжено с большими экономическими затратами. Приемлемым решением может стать напыление слоя меди поверх уже готовых дверных ручек, переключателей, держателей и др., произведенных из доступных материалов, таких как сталь, алюминий или пластик. В данной работе были оценены антибактериальные свойства алюминиевых пластин с медным напылением.
Так как основная область применения таких поверхностей – контактные поверхности в больницах, то они неизбежно будут взаимодействовать как с неорганическими загрязнителями, так и с выделениями кожи человека. В современной литературе мало обсуждается влияние загрязнений, попадающих при естественной эксплуатации, на функциональные свойства таких поверхностей касания. Чтобы восполнить этот пробел, в данной работе исследовано влияние белковых загрязнений и загрязнений, связанных с касаниями кожей человека, на антибактериальные свойства поверхностей с экстремальным смачиванием.
МАТЕРИАЛЫ И МЕТОДЫ
Для исследования влияния загрязненности поверхности на антибактериальные свойства необработанных медных (контрольных), супергидрофильных и супергидрофобных поверхностей использовали медные пластины марки М1М. Кроме того, поскольку применение массивной меди для изготовления различных медицинских изделий существенно удорожает их стоимость, для сравнения бактерицидной активности использовали алюминиевые пластины с напыленным слоем меди толщиной 100–200 мкм. Напыление производили с помощью комплекта оборудования для газодинамической металлизации ДИМЕТ модели 404 (ООО “ОЦПН”, Россия).
Лазерное текстурирование поверхностей
Для получения многомодальной шероховатости поверхности медные пластины размером 10 × 10 × 1 мм3 подвергали методу импульсной лазерной обработки, основанному на растровом сканировании поверхности материала лазерным пучком наносекундной длительности и длиной волны 1.064 мкм, длительностью импульсов 200 нс, частотой импульсов 20 кГц, и пиковой мощностью до 0.95 мДж в моде TEM00. Плотность линий составляла 400 мм–1, скорость линейного перемещения луча 100 мм/с, флюенс 0.19 Дж/м2. Луч лазера фокусировался на поверхности обрабатываемого образца в пятно с диаметром 40 мкм и двигался с помощью блока управления перемещением лазерного луча RAYLASE MS10 2 (Германия). Детальное исследование морфологии получающейся в таком процессе поверхностной текстуры было проведено в нашей недавней работе [20]. Основные характерные элементы текстуры и их размеры следующие. Макрорельеф формируют слабоупорядоченные чередующиеся гребни и впадины, сформированные крупными пористыми ветвеобразными агрегатами наночастиц, с характерным шагом между гребнями/впадинами порядка 50 мкм и глубиной впадин (толщиной пористого слоя) в интервале 1–10 мкм. Следующий уровень характерных размеров рельефа составляют более мелкие агрегаты наночастиц, формирующие “листья” ветвеобразных агрегатов, их размер порядка десятков и сотен нм, а также формирующиеся при термическом отжиге сфероидальные микрочастицы размером до нескольких мкм, густо покрытые нановискерами. К третьему уровню характерных размеров относятся упомянутые нановискеры, имеющие диаметр порядка 10 нм и длину от сотен нм до нескольких мкм, а также отдельные наночастицы, составляющие основу вышеописанных пористых агрегатов и прочно “спеченные” с ними – их характерные размеры 30–50 нм.
Описанный режим лазерной обработки приводил к формированию развитой супергидрофильной поверхности, в которую капиллярное впитывание капли исследуемой бактериальной дисперсии происходило полностью в течение нескольких секунд.
Создание супергидрофобного покрытия
Для создания супергидрофобных поверхностей полученные супергидрофильные пластины подвергали предобработке на установке Plasma Cleaner PDC-030 (Zhengzhou CY Scientific Instrument, Китай). В данной работе применяли кислородную плазму при давлении О2 – 20–30 Па. При этом поверхность меди обогащалась хемосорбционно-активными центрами, необходимыми для дальнейшей гидрофобизации.
Методика гидрофобизации ранее была детально представлена в [20], поэтому здесь мы остановимся на ней вкратце. Гидрофобизацию проводили с помощью хемосорбции из паров гидрофобного агента — метокси-{3 [(2, 2, 3, 3, 4, 4, 5, 5, 6, 6, 7, 7, 8, 8, 8-пентадекафтороктил)-окси]-пропил}-силана. Это вещество было синтезировано в лаборатории академика А.М. Музафарова. Процесс хемосорбции проводили в герметичном сосуде в печи при температуре Т = 105°С. Далее образцы с помощью УЗ-ванны отмывали в спирте и ацетоне для того, чтобы избавиться от физически адсорбированных на поверхности молекул гидрофобизатора, после чего помещали в печь для окончательной сшивки гидрофобизирующего агента при Т = 150°С.
Методы искусственного биологического загрязнения поверхностей
При практическом применении антибактериальных покрытий, используемых на поверхностях касания, возникает проблема оценки влияния биологических загрязнений, наносимых на поверхность при контакте с руками пациентов, на антибактериальную активность покрытий. Для исследования такого влияния третью часть контрольных, гидрофобных и гидрофильных пластин, а также пластин с напыленным медным покрытием контаминировали пептонным раствором на основе бактериологического пептона (RM001, HiMedia Laboratories Pvt. Ltd., Индия), вторую треть образцов каждого типа загрязняли потожировыми отложениями, присутствующими на пальцах рук. Оставшуюся третью часть образцов необработанных, супергидрофильных и супергидрофобных медных пластин и алюминиевых пластин с медным напылением использовали для сравнения бактерицидного действия исходных образцов с различным смачиванием с таковыми после биологического загрязнения. Полученные образцы были маркированы следующим образом: необработанные B, с напыленным медным покрытием С, супергидрофильные Phil, супергидрофобные Phob. Для обозначения типа биологического загрязнения к приведенным выше маркировкам добавлялись буквы P, если загрязнение осуществлялось пептонным раствором и F, если загрязнение обеспечивалось касанием пальцами рук.
Для обработки в пептонном растворе подложку с пластинами погружали в 2% пептонный раствор и выдерживали на протяжении 1 мин. Далее, подложку с пластинами вынимали из раствора и сушили в вытяжном шкафу на протяжении 10 мин. Процесс повторяли 10 раз, после последнего погружения пластины просушивали 30 мин и упаковывали в чашку Петри для транспортировки и хранения.
Загрязнение потожировыми выделениями кожи производили путем касания пластин подушечками пальцев различными людьми. Для стандартизации этой процедуры загрязнения, каждого образца касались руки 47 человек.
Все полученные пластины перед экспериментом по исследованию антибактериальной активности обрабатывали УФ излучением с длиной волны 253.7 нм и мощностью 15 Вт по 30 мин с каждой стороны, чтобы предотвратить контаминацию нелабораторными штаммами микроорганизмов.
Протоколы оценки бактерицидного действия
В данной работе исследовали бактерицидную активность медных пластин по отношению к дисперсии, содержащей клетки S. aureus (Staphylococcus aureus ATCC® 29213, Becton Dickinson, США). Для приготовления бактериальной дисперсии суточную бактериальную культуру S. aureus вносили в мясопептонный бульон (МПБ; “Медгамал”, Москва) и инкубировали при 37°C в течение 18 ч.
Инкубированную дисперсию разбавляли МПБ до оптической плотности, соответствующей бактериальному титру ~108 КОЕ/мл, при этом для контаминирования тестируемых пластин использовали капли бактериальной дисперсии объемом 10 мкл.
Необходимое в соответствии с экспериментальными протоколами (рис. 1) количество медных необработанных (контрольных), супергидрофильных и супергидрофобных пластин помещали в сухие или влажные условия. Эксперимент в сухих условиях проводили в открытой чашке Петри, при этом происходило постепенное высыхание капель бактериальной дисперсии на поверхности пластин. Эксперимент в условиях влажной среды проводили в закрытых стерильных бюксах с размещенной вдоль боковых стенок фильтровальной бумагой, смоченной физиологическим раствором. Поддержание высокой влажности препятствовало испарению воды из дисперсии и поддерживало режим “влажного” контакта дисперсия – подложка. Бактериальную загрязненность пластинки измеряли через 1.5 и 24 ч для сухого контакта, 1 и 24 ч для влажного.
Для количественной оценки бактерицидного действия после фиксированного времени контакта пластины с каплей дисперсии пластину вместе с каплей (для влажного контакта) или ее остатком (для сухого контакта) помещали в пробирку с 1 мл стерильного физиологического раствора и встряхивали на шейкере при 1050 об./мин в течение 10 мин. Далее из пробирки отбирали 0.5 мл полученной бактериальной дисперсии, готовили десятикратные разведения, из каждого разведения отбирали 0.1 мл и равномерно распределяли по поверхности чашки Петри с агаром Мюллера–Хинтона (HiMedia Laboratories Pvt. Limited, Индия). После инкубации в течение 48 ч при температуре 37°C проводили подсчет количества колоний и определяли титр бактерий на пластине. Для каждого временнóго интервала использовали отдельную пластину, и каждый эксперимент повторяли не менее трех раз.
РЕЗУЛЬТАТЫ И ОБСУЖДЕНИЕ
Влияние загрязнений на смачивание медных пластин с различной обработкой
Поскольку механизм бактерицидного действия и антибактериальная активность зависят от характера смачивания поверхностей водными средами, нами были измерены углы смачивания для исходных и биологически загрязненных пластин трех видов: контрольных, супергидрофильных и супергидрофобных. Кроме того, для исходно супергидрофобных медных пластин также были измерены и углы скатывания. Полученные результаты представлены в табл. 1
Таблица 1.
Углы смачивания различных пластин
Образец | Угол смачивания, град | Угол скатывания/соскальзывания, град |
---|---|---|
B | 71.08 ± 8.93 | – |
BP | 70.46 ± 4.15 | – |
BF | 81.17 ± 8.82 | – |
Phil | Полное растекание | – |
PhilP | Полное растекание | – |
PhilF | 59.96 ± 18.65 | – |
Phob | 170.7 ± 0.25 | 2.63 ± 1.22 |
PhobP | 142.79 ± 4.07 | 38.36 ± 8.25 |
PhobF | 133.36 ± 5.56 | 55.99 ± 15.66 |
C | 62.72 ± 11.51 | – |
CP | 31.11 ± 8.49 | – |
CF | 74.35 ± 11.38 | – |
Из представленных в табл. 1 данных следует, что наименьшее влияние биологических загрязнений на смачивание наблюдается для необработанных медных пластин. Для супергидрофильных поверхностей эффект также оказался не сильно выраженным – на поверхности, загрязненной пептонным раствором, все еще сохранялось полное растекание капли, хоть и происходило с меньшей скоростью. Некоторое ухудшение смачиваемости супергидрофильных образцов можно объяснить частичным заполнением пористой поверхности компонентами раствора. При испарении воды из пор твердая фракция, частично заполняя поры, способствует снижению шероховатости, созданию поверхности с гетерогенным смачиванием и, соответственно, более медленному растеканию капли.
Наибольший эффект обработка пептонным раствором оказала на супергидрофобную поверхность. После обработки угол смачивания уменьшился с 170.7° до 142.8°, а угол скатывания вырос с 2.6° до 38.4°. Это может быть связано с физико-химическими свойствами пептонного раствора. Снижение угла смачивания на супергидрофобных поверхностях можно объяснить тем, что образующаяся при высыхании раствора бактериологического пептона пленка содержит аминокислоты и пептиды, гидрофильные функциональные группы которых склонны к образованию водородных связей c водой, что улучшает смачивание.
После обработки потожировыми выделениями с пальцев рук у контрольных и супергидрофильных образцов угол смачивания увеличился, а у супергидрофобных – значительно уменьшился.
Для объяснения наблюдаемого изменения смачивания здесь следует отметить, что состав потожировых выделений сильно зависит от внешних условий, физической активности, температуры и множества других параметров, а также сильно изменяется для каждого отдельного человека [28–30]. На ладонях и пальцах человека находятся только эккринные потовые железы и отсутствует выработка себума – кожного сала [31]. Пот из этого типа желез в основном состоит из воды и растворенных минеральных солей – хлоридов калия, натрия и кальция, а также некоторых органических веществ – лактата, мочевины, аминокислот, аммиака.
Однако в условиях повседневной жизни неизбежно происходят контакты человеческих рук с другими поверхностями тела – лицом, волосами, на которых активно вырабатывается кожное сало – себум. Поскольку в состав себума входят гидрофобные вещества, такие как триглицериды, восковые эфиры, жирные кислоты и сквален [32], такие загрязнения, попадающие на гидрофильные и супергидрофильные образцы, должны вызывать гидрофобизацию их поверхности. Для супергидрофобных поверхностей ситуация иная. Для получения наших супергидрофобных поверхностей мы использовали фтороксисилан для снижения поверхностной энергии текстурированной металлической поверхности. Согласно литературным данным, фторированные соединения с длинной фторуглеродной цепью относятся к соединениям с наименьшей поверхностной энергией. Гидрофобные соединения, присутствующие в потожировых загрязнениях, имеют различные функциональные группы, включая как гидрофобные, так и гидрофильные, что отвечает гораздо большей поверхностной энергии, чем у фтороксисиланов. Поэтому нанесение потожировых загрязнений должно приводить к повышению поверхностной энергии супергидрофобных образцов и, как следствие, к снижению углов смачивания и повышению углов скатывания. При очень значительных загрязнениях возможна даже потеря супергидрофобного состояния поверхности. Данные, представленные в табл. 1, указывают на переход из супергидрофобного в гидрофобное состояние после 47 касаний пальцами различных людей. Такое изменение смачивания, очевидно, должно способствовать увеличению площади контакта между каплей бактериально загрязненной дисперсии и образцом и находить отражение в его антибактериальной активности.
Определение антибактериальной активности
После изучения влияния загрязнений на смачиваемость образцов были проведены опыты по определению антибактериальной активности каждого из типов исследуемых здесь материалов. Контрольные, напыленные, супергидрофильные и супергидрофобные образцы были исследованы с помощью двух протоколов (влажного и сухого контакта) при двух временных промежутках – часовом и суточном.
На чашках Петри с контрольными медными и супергидрофильными пластинами, как исходными, так и загрязненными пептонным раствором или потожировыми выделениями, роста S. aureus не наблюдали, что подтверждает высокую бактерицидную эффективность объемной меди, которая была неоднократно отмечена в литературе ранее [21, 26]. Бактериальное загрязнение пластин с напыленной медью, которое наблюдали после контакта с каплями бактериальной дисперсии, представлено на рис. 2.
Рис. 2.
Экспериментальные данные бактериальной загрязненности пластин с напыленным медным покрытием: исходных, обработанных пептонным раствором и загрязненных потожировыми выделениями. Бактериальная загрязненность возникала в результате контакта пластин с каплей бактериальной дисперсии в условиях сухого контакта (СХ) в течение либо 1.5 (1), либо 24 ч (2). Исходный титр бактериальной дисперсии 2 × 108 КОЕ/мл, то есть в капле содержалось 2 × 106 КОЕ S. aureus.
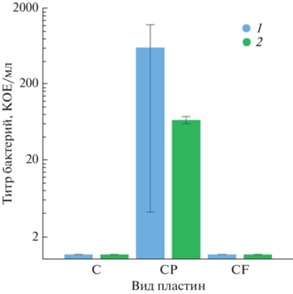
Полученные данные позволяют рассматривать напыленные медные пленки, как поверхности с высокой бактерицидной эффективностью. Даже на медных пленках, обработанных пептонным раствором, который при испарении растворителя формирует на поверхности пептонный слой, бактериальный титр не превышает 5 × 102 КОЕ/мл для исходно очень высокой концентрации бактериальных клеток, что указывает на бактерицидную эффективность таких поверхностей, равную 99.975%.
На супергидрофобных пластинах картина была более сложная. Полученные результаты отображены на рис. 3.
Рис. 3.
Экспериментальные данные бактериальной активности на супергидрофобных пластинах с различной обработкой. Исходный титр бактериальной дисперсии 9 × 109 КОЕ/мл, то есть на поверхность пластины попали 9 × 107 КОЕ. Сухой контакт 1.5 (1), 24 ч (2); влажный контакт 1 (3) и 24 ч (4).
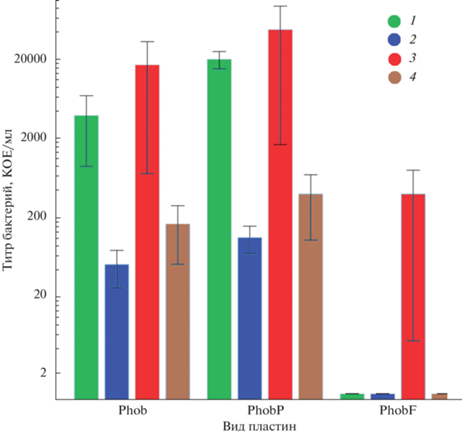
Полученные результаты можно объяснить следующим образом. На исходных супергидрофобных пластинах, имеющих очень высокие углы смачивания (170.7 ± 0.25°) и гетерогенный режим смачивания, площадь контакта капля бактериальной дисперсии/подложка оказывается очень маленькой. Это снижает вероятность непосредственного контакта бактерий с медной подложкой, а супергидрофобное состояние поверхности подавляет перенос ионов из подложки в дисперсию. Таким образом, подавляется контакт бактериальных клеток с ионами меди, которые оказывают токсическое действие на клетки S. aureus. Как было показано ранее в нашeй работe [23], ингибирование контакта ионов меди с бактериальными клетками имеет место до развития коррозионного процесса на поверхности медных пластин. Именно поэтому концентрация в капле бактериальной дисперсии на супергидрофобной подложке не снижается так же быстро, как при контакте с гладкими или супергидрофильными пластинами.
Что касается антибактериальной активности супергидрофобных образцов, контаминированных потожировыми выделениями, то, как отмечалось выше, именно при такой контаминации супергидрофобные пластины обладали наименьшими углами смачивания и наибольшими углами скатывания. Уменьшение угла смачивания приводит к увеличению площади реального контакта капли с поверхностью, что упрощает как осаждение бактериальных клеток на поверхность меди, так и переход токсичных ионов меди в жидкость. Кроме того, в состав потожирового слоя входят минеральные соли (хлориды натрия и калия), которые дополнительно интенсифицируют коррозию меди и насыщение жидкости ионами.
Для дополнительной проверки этого предположения было необходимо узнать, какое количество бактерий остается в капле бактериальной дисперсии, а какое − на поверхности супергидрофобной пластины. Для этого используемые протоколы были модифицированы следующим образом. Супергидрофобную пластину и осажденную на нее каплю по окончанию времени выдержки (1 или 24 ч) в условиях влажного контакта помещали в две разные пробирки с 1 мл физиологического раствора для раздельного встряхивания на шейкере. Далее отбираемые пробы засеивали на отдельные чашки Петри для определения количества колоний.
Через 1 ч контакта капель бактериальной дисперсии с пластинами в условиях влажного контакта растворитель практически не испарялся. Как следует из данных, представленных в табл. 2, при удалении капли поверхность оставалась бактериально чистой, поскольку не было обнаружено роста на смывах, получаемых как с исходного супергидрофобного образца, так и с супергидрофобных образцов, загрязненных пептонным раствором или потожировыми загрязнениями. При этом в объеме капель после 1 ч контакта с исходными супергидрофобными пластинами и супергидрофобными пластинами, обработанными пептонным раствором, оставалось примерно одинаковое количество колоний (около 20% от исходного значения КОЕ). При контакте капли с супергидрофобными образцами, загрязненными потожировыми выделениями, бактериальный титр оказался меньше на 3–4 порядка, что указывает на более активное бактерицидное действие подложки, связанное с развитием процессов коррозии на поверхности меди.
Таблица 2.
Результаты исследования по протоколу с разделением бактериального загрязнения капли и подложки
Тип обработки пластин | Количество бактерий через 1 ч, КОЕ | Количество бактерий через 24 ч, КОЕ | ||
---|---|---|---|---|
пластина | капля | пластина | капля | |
Phob | 0 | 3.3 × 106 | 20 | 8 × 105 |
0 | 3.3 × 106 | 0 | 2.3 × 105 | |
PhobP | 0 | 3.2 × 106 | 10 | 1.2 × 105 |
0 | 3.0 × 106 | 90 | 1.6 × 105 | |
PhobF | 0 | 102 | 0 | 0 |
0 | 4.0 × 103 | 0 | 0 | |
Исходный титр бактериальной дисперсии составлял 1.55 × 109 КОЕ/мл, то есть на поверхность пластины помещалась капля, содержащая 1.55 × 107 КОЕ. |
Через 24 ч непрерывного контакта капли с супергидрофобными подложками, ее объем значительно уменьшался за счет испарения растворителя, что приводило к аккумулированию следовых количеств бактериальных клеток на поверхности супергидрофобной меди. Высокий защитный эффект супергидрофобного покрытия на исходной супергидрофобной поверхности и поверхности, загрязненной пептонным раствором (см. табл. 1), способствовал экранированию бактериальных клеток, диспергированных в капле, от контакта с медной подложкой и ингибировал коррозионный процесс. Следствием этого является достаточно высокий титр бактериальных клеток в капле на указанных двух подложках. За 24 ч концентрация S. aureus в каплях, находящихся на обеих подложках, упала в среднем на порядок. Поверхность пластин, обработанных потожировыми выделениями, имея не очень высокий угол смачивания и высокий угол скатывания, уже не обеспечивала гетерогенный режим смачивания меди бактериальной дисперсией, что способствовало повышению бактерицидного действия меди. В результате, как в капле, так и на подложке бактерий обнаружено не было (см. табл. 2).
Таким образом, представленные в табл. 2 результаты коррелируют с влиянием загрязнений на смачивание супергидрофобных пластин: чем меньше угол смачивания и больше площадь контакта с каплей, тем легче бактериальные клетки подвергаются токсическому действию ионов меди и тем активнее взаимодействуют с опасными для клеточной оболочки наноструктурами на поверхности образцов.
Влияние загрязнений на процесс высыхания капель жидкости
Как обсуждалось выше, бактерицидная активность прямо зависит от характера смачивания поверхности меди бактериальной дисперсией. Однако после осаждения капли на твердую поверхность происходит изменение ее параметров за счет таких процессов, как испарение растворителя, взаимодействие компонентов капли с подложкой, изменение состава капли, происходящее в результате такого взаимодействия. Поэтому для детального понимания механизмов бактерицидного действия поверхностей с различным смачиванием требуется отдельное исследование изменения параметров капли при ее длительном нахождении на поверхности. Одним из основных процессов, сопровождающих длительное нахождение капли на поверхности, является ee испарение. Изменение параметров сидящей капли в процессе ее испарения в условиях недосыщения паров многократно обсуждалось в литературе [33–38].
В общем виде весь процесс испарения капли после нанесения на подложку можно описать четырьмя стадиями изменения параметров: стадия непосредственно растекания; стадия испарения с постоянным диаметром основания и уменьшающимся контактным углом; стадия испарения с постоянным контактным углом и уменьшающимся диаметром основания и, наконец, стадия испарения с уменьшением как краевого угла, так и базового диаметра до исчезновения сидящей капли. Одними из первых на существование стадий испарения с постоянным контактным углом и сжимающейся контактной линией или постоянным диаметром и одновременно контактным углом, снижающимся за счет потерь на испарение, обратили внимание Пикнетт и Бексон [33]. Авторы связали переключение со стадии постоянного диаметра на стадию постоянного угла с достижением углом смачивания значения, соответствующего углу оттекания. Позднее было показано, что на текстурированных и шероховатых подложках существование указанных стадий изменения параметров при испарении приводит к явлению залипания–проскальзывания линии трехфазного контакта капли [36, 38].
Поскольку в качестве дисперсионной среды для дисперсий S. aureus использовали фосфатный буфер (PBS), и именно дисперсионная среда, в основном, определяет процессы взаимодействия бактериальной дисперсии с супергидрофобными поверхностями [25], в данной работе мы изучили эволюцию параметров капель PBS, помещенных на все исследуемые в данной работе супергидрофобные поверхности. Были получены зависимости изменения угла смачивания, поверхностной энергии, площади контакта и объема капли фосфатного буфера (PBS) от времени нахождения капли на подложках в открытых атмосферных условиях при влажности 55.02 ± 1.12%.
На представленных на рис. 4 зависимостях изменения угла смачивания и контактного диаметра от времени можно выделить участок длительностью ~1 мин, соответствующий растеканию капли после ее помещения на поверхность. Это растекание соответствует установлению квазиравновесной формы капли, характеризуемой углом натекания. Испарение капли, происходящее в течение всего периода наблюдения за ней, приводит к постоянному снижению ee объема. На зависимости контактного диаметра от времени можно выделить и второй участок, от 1 до 6 мин, соответствующий постоянству контактного диаметра при снижении угла смачивания. Также на зависимостях угла смачивания и контактного диаметра можно отметить слабо выраженные скачки угла и диаметра на 24, 38, 42 мин, соответствующие залипанию-проскальзыванию линии трехфазного контакта капли (выделены стрелками). Интересно отметить, что поверхностное натяжение капли длительное время падает в процессе испарения, что можно связать с десорбцией гидрофобного агента, физически адсорбированного на поверхности текстурированной меди, и его переходом на границу капля–пар. И хотя количество десорбированного агента может быть мало, уменьшение площади поверхности капля–пар в процессе испарения приводит к концентрированию фторированного силана на поверхности капли и соответствующему дальнейшему падению поверхностного натяжения.
Рис. 4.
Изменение во времени характеристик капли PBS на исходной супергидрофобной пластине: (а) отношение поверхностного натяжения σ к плотности ρ; (б) диаметр контакта D, (в) угол смачивания θ; (г) объем капли V. Стрелки указывают на участки, соответствующие залипанию−проскальзыванию линии трехфазного контакта капли. Экспериментальная погрешность величин, показанных на графиках, меньше размера значков, используемых для обозначения соответствующих данных.
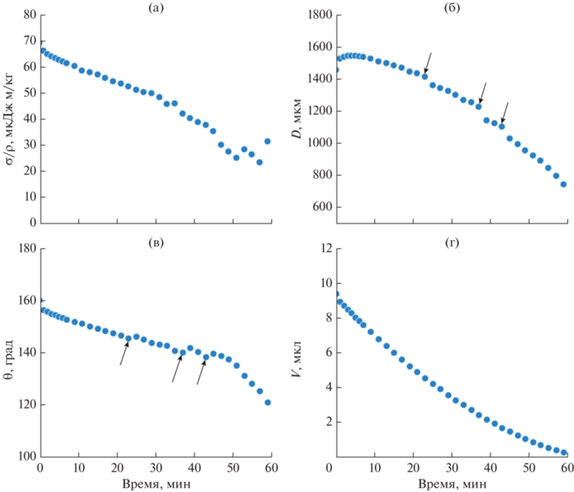
Здесь следует отметить, что в процессе испарения на исходной супергидрофобной поверхности не происходит длительного залипания линии трехфазного контакта, и вся капля стягивается в объем с очень небольшой площадью контакта с поверхностью. На это указывает тот факт, что после полного испарения фосфатного буфера на поверхности остаются лишь несколько агрегированных смешанных кристаллов, содержащих хлорид натрия, гидрофосфат натрия, хлорид калия и дигидрофосфат калия (рис. 5а).
Рис. 5.
Внешний вид сухого остатка после испарения капли фосфатного буфера на супергидрофобной поверхности: (а) исходная поверхность; (б) поверхность, загрязненная потожировыми выделениями; (в) поверхность, обработанная пептонным раствором.
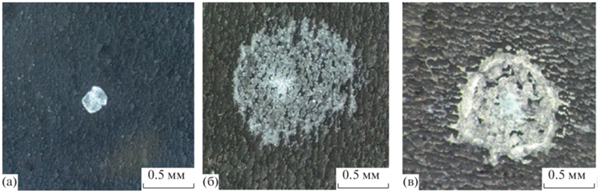
На супергидрофобной поверхности, загрязненной потожировыми выделениями (рис. 5б) и пептонным раствором (рис. 5в), сухое кристаллическое вещество фосфатного буфера после испарения капли распределено практически по всей начальной площади капли, указывая на зацепление контактной линии уже на первых этапах испарения. На это же указывает и поведение угла смачивания и контактного диаметра капли в процессе испарения (рис. 6 и 7). Небольшой рост поверхностного натяжения капли при больших временах контакта на представленных зависимостях указывает на концентрирование солей в капле при ее испарении.
Рис. 6.
Изменение во времени характеристик капли PBS на обработанной потожировыми выделениями супергидрофобной пластине: (а) отношение поверхностного натяжения σ к плотности ρ; (б) диаметр контакта D, (в) угол смачивания θ; (г) объем капли V. Экспериментальная погрешность величин, показанных на графиках, меньше размера значков, используемых для обозначения соответствующих данных.
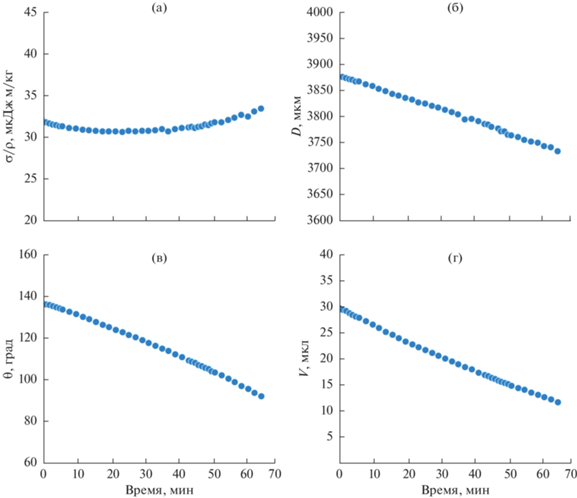
Рис. 7.
Изменение во времени характеристик капли PBS на обработанной пептонным раствором супергидрофобной пластине: (а) отношение поверхностного натяжения σ к плотности ρ; (б) диаметр контакта D, (в) угол смачивания θ; (г) объем капли V. Стрелки указывают на участки, соответствующие залипанию-проскальзыванию линии трехфазного контакта капли. Экспериментальная погрешность величин, показанных на графиках, меньше размера значков, используемых для обозначения соответствующих данных.
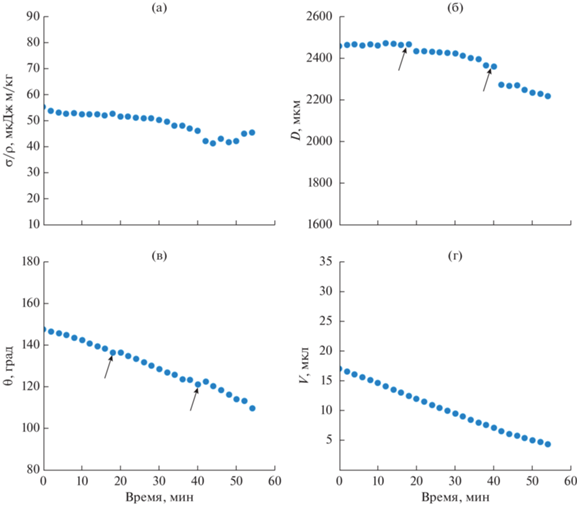
Известно, что увеличение концентрации хлоридов калия и натрия повышает величину поверхностного натяжения водных растворов. Однако, при испарении капли даже небольшого объема, концентрация солей начинает значимо повышаться только при больших временах испарения с многократным изменением объема капли. Следовательно, не ожидается значительного роста плотности раствора на исследованных временах контакта капли PBS с подложками и наблюдающихся изменениях объема капель (рис. 6 и 7). Таким образом, показанное на этих рисунках небольшое повышение отношения поверхностного натяжения к плотности раствора можно связать с влиянием соли на поверхностное натяжение капли.
Наличие участков со скачкообразным изменением контактного диаметра капли и угла смачивания (рис. 7б, 7в) указывает на залипание–проскальзывание линии трехфазного контакта при испарении капли PBS на обработанной пептонным раствором супергидрофобной пластине. Такое поведение можно интерпретировать, как зацепление линии трехфазного контакта на гидрофильных функциональных группах бактериологического пептона, осаждаемого на поверхность покрытия при контаминации пептонным раствором.
ВЫВОДЫ
В данной работе было показано, что бактерицидная эффективность как контрольных медных и супергидрофильных медных пластин, так и напыленных медных пленок оказывается близкой к 100% и практически не изменяется после загрязнения пептонным раствором или потожировыми выделениями. Это позволяет рассматривать такие материалы, как перспективные для практического применения в учреждениях, связанных с оказанием медицинской помощи. Супергидрофобные поверхности с высокими значениями углов смачивания (170.7 ± 0.25°) показывают значительно меньшую бактерицидную эффективность по отношению к клеткам, находящимся в бактериальной дисперсии, за счет малой площади контакта с каплями бактериальной культуры. Защитные свойства супергидрофобного покрытия подавляют два главных механизма бактерицидного действия медных текстурированных поверхностей – токсическое действие ионов меди и разрушение клеточных оболочек при механическом взаимодействии с наночастицами иерархической поверхности. В то же время, поскольку благодаря супергидрофобности поверхности капли бактериальных дисперсий спонтанно удаляются с поверхности при ее небольшом наклоне или под воздействием вибрации и ветровой нагрузки, супергидрофобные поверхности остаются бактериально чистыми даже при контакте с сильно бактериально загрязненными биологическими средами. В связи с тем, что супергидрофобные свойства поверхности могут быть скомпрометированы биологическими загрязнениями, попадающими на такие поверхности с рук пациентов, мы в данной работе исследовали эффект деградации бактерицидных свойств, связанный именно с такими внешними загрязнениями. В целом, представленные здесь результаты позволяют рассматривать использование медных поверхностей с различным смачиванием, как эффективный альтернативный метод в борьбе с внутрибольничными инфекциями.
Список литературы
Suksatan W., Jasim S.A., Widjaja G., Jalil A.T., Chupradit S., Ansari M.J., Mustafa Y.F., Hammoodi H.A., Mohammadi M.J. Assessment effects and risk of nosocomial infection and needle sticks injuries among patients and health care workers // Toxicology Reports. 2022. V. 9. P. 284–292. https://doi.org/10.1016/j.toxrep.2022.02.013
Nimer N.A. Nosocomial infection and antibiotic-resistant threat in the middle east // Infection and Drug Resistance. 2022. V. 15. P. 631−639. https://doi.org/10.2147/IDR.S351755
Du Q., Zhang D., Hu W., Li X., Xia Q., Wen T., Jia H. Nosocomial infection of COVID‑19: A new challenge for healthcare professionals // International Journal of Molecular Medicine. 2021. V. 47. № 4. P. 1–1. https://doi.org/10.3892/ijmm.2021.4864
World Health Organization. The burden of health care-associated infection worldwide // World Health Organization. 2010.
Ananda T., Modi A., Chakraborty I., Managuli V., Mukhopadhyay C., Mazumder N. Nosocomial infections and role of nanotechnology // Bioengineering. 2022. V. 9. № 2. P. 51. https://doi.org/10.3390/bioengineering9020051
Gold K., Slay B., Knackstedt M., Gaharwar A.K. Antimicrobial activity of metal and metal-oxide based nanoparticles // Advanced Therapeutics. 2018. V. 1. № 3. P. 1700033. https://doi.org/10.1002/adtp.201700033
Hobman J.L., Crossman L.C. Bacterial antimicrobial metal ion resistance // Journal of Medical Microbiology. 2015. V. 64. № 5. P. 471–497. https://doi.org/10.1099/jmm.0.023036-0
Psomas G. Copper (II) and zinc (II) coordination compounds of non-steroidal anti-inflammatory drugs: Structural features and antioxidant activity // Coordination Chemistry Reviews. 2020. V. 412. P. 213259. https://doi.org/10.1016/j.ccr.2020.213259
Ustundag B., Yilmaz E., Dogan Y., Akarsu S., Canatan H., Halifeoglu I., Cikim G., Aygun A.D. Levels of cytokines (IL-1β, IL-2, IL-6, IL-8, TNF-α) and trace elements (Zn, Cu) in breast milk from mothers of preterm and term infants // Mediators of Inflammation. 2005. V. 2005. № 6. P. 331–336. https://doi.org/10.1155/MI.2005.331
Vincent M., Hartemann P., Engels-Deutsch M. Antimicrobial applications of copper // International Journal of Hygiene and Environmental Health. 2016. V. 219. № 7. Part A. P. 585–591. https://doi.org/10.1016/j.ijheh.2016.06.003
Vincent M., Duval R.E., Hartemann P., Engels-Deutsch M. Contact killing and antimicrobial properties of copper. // Journal of Applied Microbiology. 2018. V. 124. № 5. P. 1032–1046. https://doi.org/10.1111/jam.13681
Grass G., Rensing C., Solioz M. Metallic Copper as an antimicrobial surface // Appl. Environ. Microbiol. 2011. V. 77. № 5. P. 1541–1547. https://doi.org/10.1128/AEM.02766-10
Dollwet H.H.A., Sorenson J.R.J. Historic uses of copper compounds in medicine // Trace Elem. Med. 1985. V. 2. № 2. P. 80–87.
Inkinen J., Makinen R., Keinanen-Toivola M.M., Nordstrom K., Ahonen M. Copper as an antibacterial material in different facilities // Lett. Appl. Microbiol. 2017. V. 64. № 1. P. 19–26. https://doi.org/10.1111/lam.12680
Arendsen L.P., Thakar R., Sultan A.H. The use of copper as an antimicrobial agent in health care, including obstetrics and gynecology // Clinical Microbiology Reviews. 2019. V. 32. № 4. P. e00125-18. https://doi.org/10.1128/cmr.00125-18
de Romaña D.L., Olivares M., Uauy R., Araya M. Risks and benefits of copper in light of new insights of copper homeostasis // Journal of Trace Elements in Medicine and Biology. 2011. V. 25. № 1. P. 3–13. https://doi.org/10.1016/j.jtemb.2010.11.004
Turnlund J.R., Keyes W.R., Kim S.K., Domek J.M. Long-term high copper intake: Effects on indexes of copper status, antioxidant status, and immune function in young men // The American Journal of Clinical Nutrition. 2004. V. 79. № 6. P. 1037–1044. https://doi.org/10.1093/ajcn/79.6.1037
Pelgrom S.M.G.J., Lock R.A.C., Balm P.H.M., Bonga S.W. Integrated physiological response of tilapia, Oreochromis mossambicus, to sublethal copper exposure // Aquatic Toxicology. 1995. V. 32. № 4. P. 303–320. https://doi.org/10.1016/0166-445X(95)00004-N
Emelyanenko A.M., Kaminsky V.V., Pytskii I.S., Emelyanenko K.A., Domantovsky A.G., Chulkova E.V., Shiryaev A.A., Aleshkin A.V., Boinovich L.B. Antimicrobial activity and degradation of superhydrophobic magnesium substrates in bacterial media // Metals. 2021. V. 11. № 7. P. 1100. https://doi.org/10.3390/met11071100
Boinovich L.B., Emelyanenko K.A., Domantovsky A.G., Chulkova E.V., Shiryaev A.A., Emelyanenko A.M. Pulsed laser induced triple layer copper oxide structure for durable polyfunctionality of superhydrophobic coatings // Advanced Materials Interfaces. 2018. V. 5. № 21. P. 1801099. https://doi.org/10.1002/admi.201801099
Emelyanenko A.M., Kaminskii V.V., Pytskii I.S., Domantovsky A.G., Emelyanenko K.A., Aleshkin A.V., Boinovich L.B. Antibacterial properties of superhydrophilic textured copper in contact with bacterial suspensions // Bulletin of Experimental Biology and Medicine. 2020. V. 168. P. 488–491. https://doi.org/10.1007/s10517-020-04737-5
Rosli N.A., Teow Y.H., Mahmoudi E. Current approaches for the exploration of antimicrobial activities of nanoparticles // Science and Technology of Advanced Materials. 2021. V. 22. №. 1. P. 885–907. https://doi.org/10.1080/14686996.2021.1978801
Emelyanenko A.M., Pytskii I.S., Kaminsky V.V., Chulkova E.V., Domantovsky A.G., Emelyanenko K.A., Sobolev V.D., Aleshkin A.V., Boinovich L.B. Superhydrophobic copper in biological liquids: Antibacterial activity and microbiologically induced or inhibited corrosion // Colloids and Surfaces B: Biointerfaces. 2020. V. 185. P. 110622. https://doi.org/10.1016/j.colsurfb.2019.110622
Zarasvand K.A., Rai V.R. Microorganisms: Induction and inhibition of corrosion in metals // International Biodeterioration & Biodegradation. 2014. V. 87. P. 66–74. https://doi.org/10.1016/j.ibiod.2013.10.023
Каминский В.В., Емельяненко А.М., Алешкин А.В., Емельяненко К.А., Бойнович Л.Б. Эффективность и механизмы бактерицидного действия на Escherichia coli супергидрофильной поверхности магниевого сплава // Микробиология. 2021. Т. 90. № 5. С. 613–617. https://doi.org/10.31857/S0026365621050098
Boinovich L.B., Kaminsky V.V., Domantovsky A.G., Emelyanenko K.A., Aleshkin A.V., Zulkarneev E.R., Kiseleva I.A., Emelyanenko A.M. Bactericidal activity of superhydrophobic and superhydrophilic copper in bacterial dispersions // Langmuir. 2019. V. 35. № 7. P. 2832–2841. https://doi.org/10.1021/acs.langmuir.8b03817
Boinovich L.B., Modin E.B., Aleshkin A.V., Emelyanenko K.A., Zulkarneev E.R., Kiseleva I.A., Vasiliev A.L., Emelyanenko A.M. Effective antibacterial nanotextured surfaces based on extreme wettability and bacteriophage seeding // ACS Applied Nano Materials. 2018. V. 1. №. 3. P. 1348–1359. https://doi.org/10.1021/acsanm.8b00090
Baker L.B., Wolfe A.S. Physiological mechanisms determining eccrine sweat composition // European Journal of Applied Physiology. 2020. V. 120. P. 719–752. https://doi.org/10.1007/s00421-020-04323-7
Verde T., Shephard R.J., Corey P., Moore R. Sweat composition in exercise and in heat // Journal of Applied Physiology. 1982. V. 53. № 6. P. 1540–1545. https://doi.org/10.1152/jappl.1982.53.6.1540
Wilke K., Martin A., Terstegen L., Biel S.S. A short history of sweat gland biology // International Journal of Cosmetic Science. 2007. V. 29. № 3. P. 169–179. https://doi.org/10.1111/j.1467-2494.2007.00387.x
Collins K.J. Composition of palmar and forearm sweat // Journal of Applied Physiology. 1962. V. 17. № 1. P. 99–102. https://doi.org/10.1152/jappl.1962.17.1.99
Picardo M., Ottaviani M., Camera E., Mastrofrancesco A. Sebaceous gland lipids // Dermato-Endocrinology. 2009. V. 1. № 2. P. 68–71. https://doi.org/10.4161/derm.1.2.8472
Picknett R.G., Bexon R. The evaporation of sessile or pendant drops in still air // Journal of Colloid and Interface Science. 1977. V. 61. № 2. P. 336–350. https://doi.org/10.1016/0021-9797(77)90396-4
Bourges-Monnier C., Shanahan M.E.R. Influence of evaporation on contact angle // Langmuir. 1995. V. 11. № 7. P. 2820–2829. https://doi.org/10.1021/la00007a076
Bennacer R., Ma X. Effect of temperature and surfactants on evaporation and contact line dynamics of sessile drops // Heliyon. 2022. V. 8. № 11. P. e11716. https://doi.org/10.1016/j.heliyon.2022.e11716
Anantharaju N., Panchagnula M., Neti S. Evaporating drops on patterned surfaces: Transition from pinned to moving triple line // Journal of Colloid and Interface Science. 2009. V. 337. № 1. P. 176–182. https://doi.org/10.1016/j.jcis.2009.04.095
Misyura S.Y., Andryushchenko V.A., Morozov V.S., Smovzh D.V. The effect of textured surface on graphene wettability and droplet evaporation // Journal of Materials Science. 2022. V. 57. № 3. P. 1850–1862. https://doi.org/10.1007/s10853-021-06853-7
Chulkova E.V., Emelyanenko K.A., Emelyanenko A.M., Boinovich L.B. Elimination of wetting study flaws in unsaturated vapors based on Laplace fit parameters // Surface Innovations. 2022. V. 10. № 1. P. 21–24. https://doi.org/10.1680/jsuin.21.00012
Дополнительные материалы отсутствуют.
Инструменты
Коллоидный журнал