Теплоэнергетика, 2024, № 3, стр. 18-31
Разработка конструктивных решений аккумулятора фазового перехода в условиях его функционирования в едином энергокомплексе с аэс
Р. З. Аминов a, *, М. В. Гариевский a, Д. М. Аношин a
a Саратовский государственный технический университет им. Ю.А. Гагарина
410054 г. Саратов, Политехническая ул., д. 77, Россия
* E-mail: oepran@inbox.ru
Поступила в редакцию 20.03.2023
После доработки 31.07.2023
Принята к публикации 30.08.2023
Аннотация
В условиях роста доли атомных электростанций в энергосистемах европейской части России и дефицита маневренных генерирующих мощностей возникает необходимость привлечения АЭС к участию в покрытии переменной части графика электрических нагрузок. Использование аккумулирующих установок, таких как тепловые аккумуляторы фазового перехода, способные запасать тепловую энергию, получаемую от реакторных установок АЭС в часы спада нагрузки в энергосистеме, и расходовать ее в часы пиковых нагрузок для выработки электроэнергии, позволит повысить системную эффективность АЭС. На основе проведенного анализа выделены перспективные теплоаккумулирующие материалы (ТАМ) с фазовым переходом для эксплуатации в системах теплового аккумулирования при температурном режиме от 200 до 300°С, который обусловлен характеристиками паротурбинной установки АЭС, в том числе параметрами питательной воды и свежего пара. Для принятой схемы установки с аккумулятором фазового перехода (АФП) с повышением температуры питательной воды после подогревателей высокого давления двухконтурной АЭС разработаны методологические основы выбора конструктивных решений системы аккумулирования с нитратом лития в качестве теплоаккумулирующего материала. Методом конечных элементов в программном комплексе для ЭВМ проведено моделирование нестационарного теплообмена между этим материалом и водой для оребренных и неоребренных труб применительно к элементарной секции АФП. По результатам расчетов построены графики зависимости тепловой мощности секции от продолжительности разрядки АФП. Предложены способы расчета продолжительности разрядки АФП и массы необходимого теплоаккумулирующего материала при снижении тепловой мощности. Для схемы с дополнительной паротурбинной установкой мощностью 12 МВт (для энергоблоков АЭС с ВВЭР-1200) определены основные характеристики аккумулятора фазового перехода и эффективность предложенного решения при различной продолжительности разрядки АФП.
Увеличение доли атомных электростанций в структурах энергосистем европейской части России при наблюдаемом дефиците маневренных генерирующих мощностей приводит к необходимости привлечения АЭС к участию в покрытии переменной части графика электрических нагрузок. В этих условиях возрастает потребность в энергоаккумулирующих установках. К их числу можно отнести тепловые аккумуляторы фазового перехода, которые способны запасать тепловую энергию, полученную от реакторных установок АЭС в часы спада нагрузки в энергосистеме, и использовать ее в часы пиковых нагрузок для выработки электроэнергии, что позволит повысить системную эффективность АЭС [1–7]. Производство электроэнергии в часы повышенной электрической нагрузки может увеличить прибыль атомной станции и обеспечить окупаемость системы аккумулирования тепловой энергии.
В проведенных ранее исследованиях [7–9] по интеграции АФП в тепловую схему двухконтурной АЭС отмечена высокая эффективность такого решения. Для принятой авторами схемы с дополнительной паровой турбиной мощностью 12 МВт появляется возможность осуществлять непрерывное электроснабжение собственных нужд АЭС при наступлении аварийной ситуации с обесточиванием благодаря использованию пара, генерируемого с помощью остаточного тепловыделения остановленного реактора. Это позволит повысить уровень безопасности АЭС в соответствии с возрастающими требованиями Международного агентства по атомной энергии и обеспечить работу энергоблока в режиме регулирования графика электрических нагрузок без изменения мощности реакторной установки. Расчеты накопленного чистого дисконтированного дохода с учетом многофункциональных свойств разрабатываемой схемы показали, что он имеет положительное значение во всем принятом диапазоне разности дневного и ночного тарифов на электроэнергию [7]. Таким образом, предложенная схема с дополнительной паровой турбиной мощностью 12 МВт дает возможность значительно повысить маневренность и конкурентоспособность АЭС.
Использование скрытой теплоты фазового перехода теплоаккумулирующего материала – эффективный и перспективный способ хранения энергии [10–15]. Аккумулирующие системы позволяют запасать тепловую энергию за счет скрытой теплоты фазового перехода при высокой плотности ее накопления и изменении агрегатного состояния самого материала. При этом обеспечивается почти постоянная температура зарядки/разрядки АФП. Именно поэтому аккумулирование тепловой энергии с фазовым переходом является одной из основных тем исследований в последние 30 лет [16–22].
Выбор материала с фазовым переходом для систем АФП зависит от его свойств, емкости системы, рабочей температуры, производительности и конструкций теплообменников. Характеристики аккумулятора фазового перехода и свойства теплоаккумулирующих материалов взаимосвязаны и существенно влияют друг на друга. К системе хранения тепла предъявляются следующие требования:
большая емкость накопления энергии на единицу объема;
соответствие температуры плавления параметрам технологических процессов в интегрируемой электростанции;
хороший теплообмен между теплоносителем и аккумулирующей средой;
высокая механическая и химическая стабильность аккумулирующих материалов;
хорошая совместимость между теплоносителем, теплообменником и/или теплоаккумулирующим материалом (безопасность);
полная обратимость в течение многих циклов зарядки/разрядки (длительность срока службы);
минимальные тепловые потери;
простота управления режимами.
Кроме того, очень важны низкие капиталовложения в системы хранения тепловой энергии и небольшие затраты на их техническое обслуживание [23].
Для реальных установок трудно найти идеальный материал, отвечающий всем перечисленным условиям одновременно. Соответствие температуры фазового перехода параметрам установки, высокая удельная теплота фазового перехода и низкая стоимость являются главными требованиями к теплоаккумулирующему материалу. Если они выполняются, тогда всесторонне рассматриваются другие факторы.
Хотя системы накопления тепловой энергии на основе теплоты фазового перехода имеют преимущества по сравнению с обычной системой теплового аккумулирования, они также обладают недостатками, которые ограничивают их широкое применение. Далее указаны эти недостатки и рассмотрены проблемы, связанные с системой хранения тепловой энергии с помощью теплоаккумулирующих материалов с фазовым переходом [24].
Самая большая технологическая проблема реализации системы хранения на основе АФП – низкая теплопроводность теплоаккумулирующего материала, которая, как правило, не превышает 0.7 Вт/(м ⋅ К). Плавление ТАМ происходит обычно намного быстрее, чем его затвердевание, что связано с эффектом плавучести во время плавления, увеличивающим передачу тепла вследствие конвективного теплообмена. При разрядке АФП энергия, выделяющаяся в процессе затвердевания аккумулирующего материала, должна передаваться от границы раздела твердое тело – жидкость через растущий твердый слой к поверхности теплообменника. При этом большое значение имеет теплопроводность твердого ТАМ.
Еще одним важным фактором, ограничивающим широкое использование систем аккумулирования тепловой энергии на основе АФП, является количество циклов, которые выдерживает теплоаккумулирующий материал без ухудшения свойств. Долговременная стабильность системы хранения ТАМ может быть нарушена из-за слабой стабильности самого материала при интенсивном термическом циклировании и/или коррозии либо химической несовместимости между ТАМ и материалом конструкций АФП.
Следующая проблема – переохлаждение, которое препятствует выделению скрытой теплоты фазового перехода при достижении точки кристаллизации ТАМ в процессе разряда. Переохлаждение происходит в момент, когда температура материала с фазовым переходом в жидком состоянии оказывается ниже точки кристаллизации без затвердевания, а сам материал остается в метастабильном состоянии, при котором скрытая теплота плавления не выделяется. Причиной переохлаждения является либо низкая скорость образования центров кристаллизации, либо невысокая скорость кристаллизации, либо оба эти фактора вместе. Переохлаждение на несколько градусов нарушает процесс отбора тепла и может полностью его остановить.
Еще один минус систем аккумулирования тепловой энергии на основе АФП – их высокая стоимость: из-за недостаточной теплопроводности ТАМ должны быть применены эффективные технологии, улучшающие теплопередачу, реализация которых приводит к увеличению затрат.
И, наконец, последний недостаток таких систем – изменение объема ТАМ во время фазового перехода твердое тело – жидкость.
Таким образом, разработка тепловых аккумуляторов на основе материалов с фазовым переходом, которые обладают улучшенными теплофизическими свойствами и стабильными характеристиками при низкой стоимости, а также оптимизация конструкции теплообменника и исследование режимов его работы являются главными задачами при совершенствовании системы аккумулирования тепла со среднетемпературными материалами с фазовым переходом (200–300°С).
ВЫБОР ТЕПЛОАККУМУЛИРУЮЩЕГО МАТЕРИАЛА
Выбор теплоаккумулирующего материала существенно зависит от условий работы соответствующей установки. Среднетемпературные расплавы солей и солевые смеси, используемые в качестве теплоаккумулирующих веществ, обычно относятся к нитратам, хлоридам, карбонатам и их эвтектическим смесям. В настоящее время основное внимание уделяется нитратам и нитритам щелочных металлов и их смесям (большинство нитратов имеют температуру плавления 200–300°С и теплоту фазового перехода 100–200 кДж/кг). Преимущества этих материалов – высокие значения рабочей температуры, удельной теплоемкости, коэффициента конвективной теплопередачи в жидком состоянии, а также термическая стабильность, малая вязкость и низкая цена. Таким образом, эти материалы считаются потенциально хорошими теплоаккумулирующими материалами. Как правило, соли лития самые дорогие, соли калия и натрия дешевле, а самую низкую цену имеют соли кальция [23].
В реальных системах для передачи или хранения тепла могут использоваться многокомпонентные составы, образованные бинарными и/или тройными эвтектическими солями. К плюсам смешанных солей относится возможность достижения желаемой температуры плавления. Кроме того, дорогие соли и солевые композиции с хорошими характеристиками аккумулирования тепла можно применять вместе с недорогими солями, чтобы снизить стоимость составов при сохранении примерно той же способности аккумулирования тепла.
Проведенный анализ литературных источников [25–32] позволил выделить перспективные теплоаккумулирующие материалы с фазовым переходом для эксплуатации в системах теплового аккумулирования с температурным режимом от 200 до 300°С в соответствии с параметрами питательной воды и свежего пара двухконтурных АЭС. В качестве теплоаккумулирующего материала, нагреваемого теплом, выделяющимся при конденсации свежего пара в часы спада электропотребления, и отдающего тепло при нагреве поступающей питательной воды основного контура регенерации в часы пиковой нагрузки, можно использовать эвтектику из нитрата натрия и гидроксида натрия (18.5 мол. % NaNO3 + 81.5 мол. % NaOH)22, а также нитрат лития LiNO3 с теплофизическими свойствами, представленными в табл. 1.
Таблица 1.
Свойство ТАМ | LiNO3 | 18.5% NaNO3 + 81.5% NaOH |
---|---|---|
Температура плавления (кристаллизации), °C | 253 (253) | 257 (254) |
Удельная теплота фазового перехода, кДж/кг | 370 | 292 |
Плотность, кг/м3 | 2310/1776 | 2150/1910 |
Удельная теплоемкость, кДж/(кг · К) | 1.800/2.030 | 1.720/2.050 |
Теплопроводность, Вт/(м · К) | 1.370/0.619 | 0.7/0.6 |
Стоимость, дол/кг | 0.67–4.00 | 0.52 |
Такой выбор материалов обусловлен отличными значениями теплофизических показателей, хорошей химической стабильностью и невысокой коррозионной активностью к металлам. Так, согласно результатам экспериментов, проведенных в Электротехнической лаборатории в Японии (Electrotechnical Laboratory, Ibaraki), бинарная эвтектическая смесь 18.5% NaNO3 + 81.5% NaOH в сравнении с другим смесями NaOH с NaN-O3 и NaOH с NaNO2 (рассматривались два соединения NaNO3$\centerdot $NaOH и NaNO3$\centerdot $2NaOH, три эвтектические смеси, состоящие из NaNO3 и NaOH в соотношениях 72 и 28, 41 и 59, 18.5 и 81.5% соответственно, одно соединение NaNO2$\centerdot $NaOH и две эвтектические смеси, состоящие из NaNO2 и NaOH в соотношениях 80 и 20, 27 и 73% соответственно) является наиболее перспективным материалом в диапазоне рабочих температур 230–300°С для аккумулирования тепловой энергии на атомных электростанциях. Такой вывод сделан потому, что эта бинарная смесь имеет большу́ю теплоту фазового перехода и низкую склонность к переохлаждению, а также хорошую стабильность после испытания в течение 1000 циклов [28].
Нитраты щелочных металлов вместе с металлическими частями АФП образуют коррозионную систему. При этом при сочетании ТАМ и конструктивных элементов АФП необходимо учитывать возможные факторы, влияющие на скорость коррозии металла: материал теплообменника, способ обработки поверхностей, тип ТАМ, наличие примесей, стабильность ТАМ, температурный режим работы. В [33] показано, что в результате испытаний на коррозию наилучшую совместимость с солями натрия имеют углеродистые и никельсодержащие стали.
Для расчета АФП принят теплоаккумулирующий материал нитрат лития (LiNO3) ввиду более высокой удельной теплоты фазового перехода среди рассмотренных материалов, что позволит ему запасать необходимое количество энергии при меньших массе ТАМ и размерах АФП. Недостатками нитрата лития являются его высокая стоимость и значительное изменение объема при фазовом переходе. На рис. 1 представлен график зависимости свойств LiNO3 от температуры.
Рис. 1.
Изменение плотности (а), удельной теплоемкости (б), теплопроводности (в) и динамического коэффициента вязкости (г) нитрата лития LiNO3 в зависимости от температуры (по данным Material Properties Database, http://www.jahm.com)
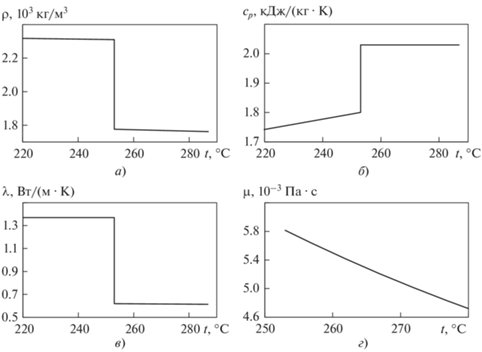
Скорости зарядки и разрядки теплового аккумулятора фазового перехода сдерживаются низкой теплопроводностью имеющихся материалов фазового перехода [от 0.1 до 0.8 Вт/(м · К)] и не могут удовлетворить потребности систем АФП. Существует несколько способов улучшить теплопередачу, чтобы увеличить скорость плавления и затвердевания. Многие исследователи изучали кожухотрубную конструкцию (более 70% работ, посвященных АФП), которая считается наиболее популярной установкой, поскольку имеет минимальные тепловые потери и легко интегрируется в систему АФП. К способам улучшения теплоотдачи кожухотрубной установки АФП относятся: оребрение труб, добавление высокотеплопроводных инклюзивов, погружение пористого материала в объем ТАМ, использование нескольких ТАМ и др. [34–37].
Увеличение площади теплопередачи при добавлении ребер – простой и эффективный способ повысить теплопередачу от ТАМ к теплоносителю [38–42]. Обнаружено, что число ребер (или шаг) и их длина оказывают большее влияние на производительность системы хранения тепла, чем их толщина и форма. В то же время выполнение радиальных ребер при вертикальной установке труб уменьшает конвекцию, вызванную плавучестью ТАМ, которая играет важную роль в процессе фазового перехода [43, 44]. Поэтому противоречие между повышением эффективной теплопроводности и одновременным подавлением эффекта плавучести должно учитываться путем выбора оптимального положения и ориентации ребер.
Диспергирование наночастиц с высокой теплопроводностью в теплоаккумулирующем материале и его погружение в пористый материал для получения композитного ТАМ является эффективным способом увеличения теплопередачи.
Тем не менее, оба эти способа могут привести к росту производственных затрат и уменьшению плотности накопления тепла. Поэтому очень важно изучить методы улучшения теплопередачи, которые не связаны со сложной обработкой поверхности теплообмена, обеспечивают неизменную плотность накопления тепла и не требуют увеличения затрат.
МОДЕЛИРОВАНИЕ НЕСТАЦИОНАРНЫХ ПРОЦЕССОВ ПРИ РАЗРЯДКЕ АФП
В силу нестационарности процессов теплообмена, протекающих при зарядке и разрядке как в теплоаккумулирующем материале, так и в теплоносителе, задача расчета процессов теплопереноса сильно усложняется. В работе [45] рассматриваются физическая и математическая модели упрощенных процессов зарядки и разрядки ячейки АФП в виде дифференциальных уравнений нестационарной теплопроводности для стенки трубы и энергии для теплоаккумулирующего материала. Проинтегрировать эти уравнения практически невозможно. По этой причине все расчеты проводятся в компьютерных программных комплексах по конечно-разностным уравнениям итерационными методами путем разбиения временнóго и геометрического пространств на отдельные подзоны. По такому принципу строятся все современные программы расчетов теплообмена в аккумуляторах фазового перехода [46–49].
Наибольший интерес при конструировании систем аккумулирования представляет разрядка АФП. Для этого процесса авторами определялись расчетные параметры теплоносителя и необходимая масса ТАМ для подогрева питательной воды с целью генерировать повышенное количество пара в основных парогенераторах при установке дополнительной паровой турбины мощностью 12 МВт [7, 8]. При таком уровне мощности может быть обеспечено аварийное резервирование собственных нужд двух энергоблоков при аварийном обесточивании АЭС. Мощность такой паровой турбины и продолжительность ее работы могут иметь и более высокие значения, которые зависят от принятой массы теплоаккумулирующего материала. Так, для энергоблока с ВВЭР-1000 мощность такой турбины может достигать 140 МВт [7].
Исходя из этого при участии одного из авторов настоящей статьи (Р.З. Аминова) была разработана и запатентована структурная схема комбинирования двухконтурной АЭС с ВВЭР с системой теплового аккумулирования (рис. 2), включающей в себя аккумулятор фазового перехода и маломощную многофункциональную паротурбинную установку [50].
Рис. 2.
Схема комбинирования энергоблока АЭС с ВВЭР и системы теплового аккумулирования с подогревом воды в АФП. 1 – реактор; 2 – парогенератор; 3 – АФП; 4 – основная паровая турбина; 5 – дополнительная паровая турбина; 6, 7 – регенеративные подогреватели низкого и высокого давления соответственно; СС – сепаратор; ПП – пароперегреватель
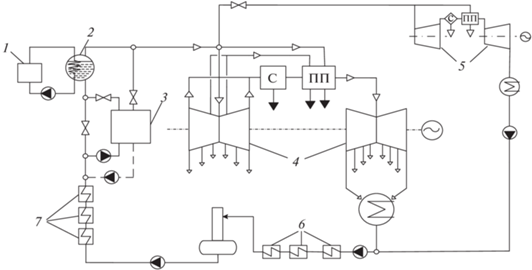
В ночные часы спада электрической нагрузки часть свежего пара из парогенераторов направляется на зарядку АФП. В режиме пиковых нагрузок при разрядке аккумулятора часть питательной воды после подогревателей высокого давления поступает в АФП и после подогрева подмешивается к питательной воде в основном тракте. Из-за повышения температуры питательной воды на входе в парогенераторы при той же мощности реактора генерируется избыток свежего пара, который направляется на дополнительную многофункциональную ПТУ.
Аккумулятор фазового перехода представляет собой кожухотрубный теплообменник с вертикальным исполнением труб и шахматным расположением трубного пучка. Расчет производился для одной ячейки АФП разной длины как с гладкими трубами, так и с трубами с дисковым поперечным оребрением. Элементы конструкции а-ккумулятора фазового перехода и трубы с оребрением показаны на рис. 3, параметры моделируемой элементарной ячейки АФП при длине трубы 1 м представлены в табл. 2.
Рис. 3.
Элементы конструкции аккумулятора фазового перехода (вид сверху). 1 – вода; 2 – труба; 3 – радиальное оребрение; 4 – граница элементарной ячейки АФП с теплоаккумулирующим материалом. Диаметр трубы внешний ${{d}_{{{\text{внеш}}}}}$ = 29 мм, внутренний ${{d}_{{{\text{внут}}}}}$ = 25 мм, диаметр ребра ${{d}_{{{\text{реб}}}}}$ = 48 мм
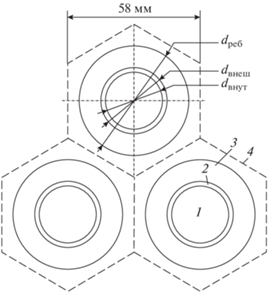
Таблица 2.
Параметры элементарной ячейки АФП с площадью основания 2913 мм2 при длине трубы 1 м
Параметр | Гладкая труба | Труба с оребрением |
---|---|---|
Площадь поверхности теплообмена с ТАМ, мм2 | 90 992 | 317 346 |
Общий объем ячейки АФП, м3 | 2.913 × 10–3 | 2.913 × 10–3 |
В том числе: | ||
ТАМ | 2.253 × 10–3 | 2.026 × 10–3 |
труба (с оребрением) | 0.170 × 10–3 | 0.397 × 10–3 |
вода | 0.491 × 10–3 | 0.491 × 10–3 |
Доля объема ТАМ в ячейке, % | 77.3 | 69.5 |
Масса ТАМ в ячейке, кг | 5.222 | 4.696 |
Энергия фазового перехода ТАМ, кДж | 1932.1 | 1737.6 |
Суммарная энергия ТАМ с учетом перегрева (272°С) и переохлаждения (220°С), кДж | 2438.7 | 2193.2 |
РЕЗУЛЬТАТЫ РАСЧЕТА ТЕМПЕРАТУРЫ ТЕПЛОНОСИТЕЛЯ НА ВЫХОДЕ ИЗ АФП
Для расчета температуры теплоносителя на выходе из АФП в ANSYS Fluent методом конечных элементов проведено математическое моделирование нестационарного теплообмена между теплоаккумулирующим материалом и водой через металлическую трубу.
В качестве материала труб принята углеродистая сталь марки 10. Зависимость теплофизических свойств стали от температуры принималась линейной по справочным данным [при температурах 200 и 300°C плотность равна 7800 и 7765 кг/м3, теплоемкость – 532 и 565 Дж/(кг · К), теплопроводность – 53.0 и 49.6 Вт/(м · К) соответственно]. Зависимость теплофизических свойств воды в трубах от температуры (при давлении 6.91 МПа) задавалась полиномами. В качестве начальных условий для решения были выбраны температуры теплоносителя на входе в АФП ${{t}_{{{\text{вх}}}}}$ = 220°С (температура питательной воды) и температура перегретого ТАМ (после нагрева свежим паром при зарядке АФП) ${{t}_{{{\text{ТАМ}}}}}$ = 272°С. Расчет выполнялся при расходах теплоносителя через элементарную ячейку АФП 0.023 и 0.01 кг/с.
Результаты моделирования разрядки элементарной ячейки АФП приведены на рис. 4.
Рис. 4.
Температуры (выделены изотермы) в сечении элементарной ячейки длиной 0.5 м при расходе воды 0.023 кг/с в различные моменты времени при разрядке АФП. Время, мин: а – 1; б – 30; в – 60
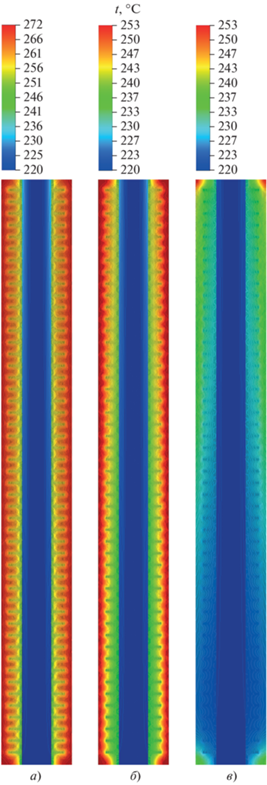
Итоги расчета температуры воды (средней по потоку) на выходе из трубы элементарной ячейки с аккумулятором фазового перехода при разрядке АФП в зависимости от времени разрядки для труб разной длины и расходов воды представлены на рис. 5.
Рис. 5.
Изменение температуры воды на выходе из ячейки при разрядке АФП для неоребренных (а, в) и оребренных (б, г) труб. Расход воды, кг/с: а, б – 0.023; в, г – 0.010. Длина трубы, м: 1 – 0.5; 2 – 1.0; 3 – 1.5; 4 – 2.0
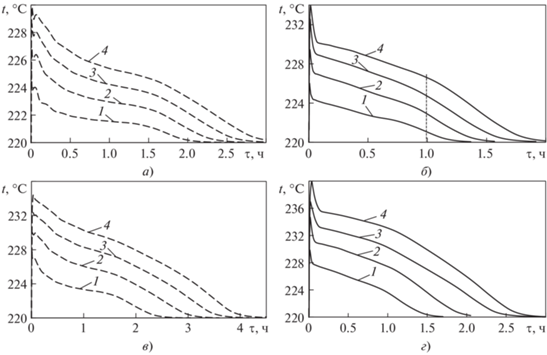
Анализируя полученные графики, можно сделать вывод, что наибольшая продолжительность разрядки характерна для гладких труб, при этом максимум температуры, как и средняя температура теплоносителя на выходе в процессе работы АФП, значительно ниже, чем для оребренных труб. При использовании гладких труб длительность разрядки аккумулятора возрастает в 1.5 раза, однако снижение средней температуры приводит к необходимости повышать загрузку теплоаккумулирующего материала и, как следствие, росту массогабаритных параметров АФП. При использовании оребренных труб ввиду увеличения поверхности теплообмена (коэффициент оребрения составляет 3.5) средний тепловой поток, воспринимаемый теплоносителем, значительно возрастает, что способствует повышению температуры на выходе из АФП.
Кроме того, оребрение позволяет равномернее охладить теплоаккумулирующий материал. Благодаря ребрам тепло отводится с большего объема теплоаккумулирующего материала и, соответственно, кристаллизация ТАМ и его остывание распределяются почти по всему объему АФП, в отличие от гладких труб, при использовании которых фронт кристаллизации медленно распространяется от центра (трубы) к периферии элементарной ячейки, из-за чего скорость кристаллизации остается довольно низкой.
Необходимо учитывать, что центры кристаллизации образуются на неровностях поверхности. Ребро также будет являться центром начала кристаллизации, что приведет к росту ее скорости. Таким образом, оребрение позволяет снизить влияние переохлаждения на работу АФП.
Главная задача аккумулирующей системы – покрытие пиковых нагрузок. Ввиду наличия гладких труб в АФП можно использовать его как дополнительный подогреватель при целесообразных габаритных размерах, но основные задачи при этом будут решены не полностью или не выполнены вовсе, что экономически не выгодно. При применении оребренных труб появляется возможность в сжатые сроки получить больше тепловой энергии и увеличить тепловую мощность аккумулирования при сокращении времени разрядки. Это позволяет покрывать пиковые нагрузки, которые длятся в среднем 1–2 ч, при этом габаритные размеры самого аккумулятора и его стоимость остаются в экономически приемлемых пределах. При аварийном обесточивании АЭС дополнительная паровая турбина продолжает работать благодаря использованию пара, генерируемого за счет остаточного энерговыделения реактора. Таким образом обеспечивается аварийное резервирование собственных нужд АЭС.
ОЦЕНКА ПРОДОЛЖИТЕЛЬНОСТИ РАБОЧЕЙ РАЗРЯДКИ АФП И КОНСТРУКТИВНЫХ ПАРАМЕТРОВ АФП
На рис. 6 показано, что тепловая мощность АФП при его разрядке зависит от продолжительности разрядки и снижается по мере затвердевания теплоаккумулирующего материала. При этом для оценки номинального уровня тепловой мощности АФП, соответствующей мощности установленной дополнительной турбины, необходимо выбрать рабочую точку ${{\tau }_{{\text{р}}}}$ на кривых разрядки, аналогичных кривым, приведенным на рис. 5, б (для оребренных труб и принятого расхода воды 0.023 кг/с). Выбранная расчетная точка будет определять необходимую загрузку ТАМ и рабочую продолжительность разрядки АФП, после которой мощности разрядки и паровой турбины начнут снижаться. Эта точка и будет соответствовать окончанию разрядки и отключению АФП. В этом случае некоторая часть аккумулированного тепла (остаточная) ${{Q}_{{{\text{ост}}}}}$ будет не востребована и должна храниться в АФП до следующего процесса зарядки. Это количество тепла будет определяться площадью под кривой разрядки справа от принятого значения времени работы АФП ${{\tau }_{{\text{р}}}}$ на рис. 5, б. Таким образом, общее количество запасаемой тепловой энергии Q, необходимой для отпуска тепла потребителю ${{Q}_{{{\text{от}}}}}$, должно составлять $Q = {{Q}_{{{\text{от}}}}} + {{Q}_{{{\text{ост}}}}}$. При смещении выбранной рабочей точки ${{\tau }_{{\text{р}}}}$ в сторону увеличения продолжительности работы (вправо на рис. 5, б) требуемая проектная загрузка ТАМ в АФП будет возрастать.
Рис. 6.
Изменение тепловой мощности АФП из 52 263 ячеек с ТАМ LiNO3 и оребренными трубами длиной 2 м в процессе разрядки
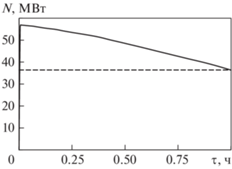
Следует определить конструктивные параметры АФП для подогрева питательной воды с целью генерировать дополнительное количество пара в основных парогенераторах АЭС для привода дополнительной паровой турбины мощностью 12 МВт в течение 1 ч.
Необходимая тепловая мощность АФП (для подогрева части питательной воды) для работы такой турбины с КПД 33% составит 36.4 МВт. Результаты расчета параметров АФП при расходе питательной воды через элементарную ячейку 0.023 кг/с при различных длинах оребренных труб приведены в табл. 3. Согласно представленным данным, при коротких трубах тепловая мощность ячейки АФП через 1 ч значительно падает, и, чтобы создать мощность АФП 36.4 МВт, необходимо существенно увеличить число ячеек. В этом случае невозможно обеспечить расчетный расход воды через одну ячейку (суммарный расход через АФП будет превышать расход питательной воды). Для АФП с элементарными ячейками высотой 2 м доля питательной воды, направляемой в АФП, составит 66%, график изменения мощности АФП в течение 1 ч в большей степени будет приближен к горизонтальному (см. рис. 6), а масса ТАМ для рассмотренных АФП будет наименьшей. Через 1 ч работы такого АФП остаточная часть аккумулированного тепла составляет ${{Q}_{{{\text{ост}}}}}$ = 26.8%, средняя температура ТАМ соответствует 244.8°C при доле жидкой фракции 17.3%. Площадь основания АФП равна 152 м2 (диаметр цилиндрического АФП – 13.9 м), масса АФП (заполненного водой) – 848 т, в том числе масса ТАМ – 490.4 т.
Таблица 3.
Результаты расчета параметров АФП в составе энергоблока АЭС
Параметр | Длина трубы, м | |||
---|---|---|---|---|
0.5 | 1.0 | 1.5 | 2.0 | |
Тепловая энергия, отпущенная от ячейки за 1 ч, кДж | 1078.5 | 1986.9 | 2747.1 | 3337.1 |
Доля энергии в остановленном АФП через 1 ч работы, % | 5.4 | 12.9 | 19.7 | 26.8 |
Мощность ячейки АФП через 1 ч, Вт | 109.0 | 303.8 | 495.9 | 695.8 |
Число ячеек | 333 730 | 119 711 | 73 327 | 52 263 |
Общая площадь поверхности теплообмена с ТАМ в АФП, тыс. м3 | 53.0 | 38.0 | 34.9 | 33.2 |
Тепловая энергия, отпущенная от АФП за 1 ч, ГДж | 359.9 | 237.9 | 201.4 | 174.4 |
Доля питательной воды, направляемой в АФП, % | – | – | 93 | 66 |
Площадь основания АФП, м2 | 972 | 349 | 214 | 152 |
Диаметр АФП, м | 35.2 | 21.1 | 16.5 | 13.9 |
Масса, т: | ||||
ячейки (ТАМ, металл, вода) | 4.099 × 10–3 | 8.198 × 10–3 | 12.297 × 10–3 | 16.417 × 10–3 |
АФП | 1367.9 | 981.4 | 901.7 | 858.0 |
ТАМ в АФП | 783.6 | 562.2 | 516.5 | 490.4 |
Стоимость ТАМ (LiNO3), руб/кг* | 50–300 | 50–300 | 50–300 | 50–300 |
Стоимость ТАМ, млн руб. | 39.4–235.1 | 28.3–168.7 | 26.0–155.0 | 24.6–147.1 |
Для выбранного расхода воды через ячейку АФП 0.023 кг/с при длине оребренной трубы 2 м (см. рис. 5, б) нагрев части питательной воды, направляемой в АФП, в первую минуту разрядки АФП составляет 14°С (до 234°С), со второй минуты он равен 10°С (до 230°С) и в конце первого часа – 6.6°С (до 226.6°С) (см. рис. 5, б). Доля питательной воды, направляемой в АФП, соответствует 66% (см. табл. 3). Таким образом, температура питательной воды после смешения потоков (на входе в парогенератор) возрастет на 9.2°С в первую минуту разрядки АФП и на 4.4°С в конце первого часа.
При увеличении длины трубы либо последовательном размещении секций можно повысить продолжительность разрядки АФП.
ЭКОНОМИЧЕСКОЕ ОБОСНОВАНИЕ ПРЕДЛОЖЕННОГО ТЕХНИЧЕСКОГО РЕШЕНИЯ
Расчет эффективности АФП при использовании его в схеме АЭС проведен по методике, представленной в [7–9]. Оценка полной стоимости АФП включала стоимость теплоаккумулирующего материала, металлической конструкции корпуса с теплоизоляцией, теплообменной поверхности с распределительными коллекторами, дополнительной вспомогательной аппаратуры (технологического оборудования, измерительных устройств, запорной арматуры и пр.), доставки составных частей до места эксплуатации, монтажа всех технических узлов с последующим пуском (приняты как стоимость корпуса и теплообменников). Ставка дисконтирования была выбрана в размере 10%. Горизонт расчета соответствовал 25 годам.
Результаты расчетов эффективности дополнительной паровой турбины и АФП различной емкости и, соответственно, с разным временем разрядки приведены в табл. 4.
Таблица 4.
Результаты расчетов технико-экономических показателей использования АФП на АЭС
Показатель | Время разрядки АФП, ч | |||
---|---|---|---|---|
1 | 8 | 12 | 16 | |
Объем АФП, м3 | 304 | 2432 | 3648 | 4864 |
Масса ТАМ в АФП, т | 490.4 | 3923.2 | 5884.8 | 7846.4 |
Стоимость, млн руб.: | ||||
ТАМ* | 24.6 | 197.1 | 295.7 | 394.3 |
корпуса с теплоизоляцией | 1.5 | 10.3 | 15.0 | 19.7 |
труб с коллекторами | 25.6 | 169.0 | 244.1 | 317.0 |
Капиталовложения в систему теплового аккумулирования, млн руб., всего | 849 | 1421 | 1731 | 2034 |
В том числе: | ||||
в АФП | 79 | 556 | 814 | 1067 |
в ПТУ | 550 | 550 | 550 | 550 |
в модернизацию электрохозяйства и систему управления | 78 | 78 | 78 | 78 |
в строительство помещения | 142 | 237 | 289 | 339 |
Снижение выручки от продажи “ночной” (“провальной”) электроэнергии, млн руб/год | 4 | 33 | 49 | 65 |
Выручка от продажи дополнительной “пиковой” электроэнергии, млн руб/год | 16 | 124 | 187 | 249 |
Дополнительная выручка, млн руб/год | 11 | 92 | 138 | 184 |
Чистый дисконтированный доход, млн руб. | –714 | –238 | 51 | 346 |
Экономическая эффективность использования на АЭС аккумулирующей системы с АФП зависит от большого числа факторов, в том числе и внешних.
Если не учитывать многофункциональные свойства дополнительной паровой турбины, то определяющим фактором эффективности ее работы при разрядке АФП является разность цен на электроэнергию, отпускаемую от АЭС в пиковые и ночные часы.
Результаты расчетов показали, что при текущем различии в ценах на электроэнергию в ночные и пиковые часы на рынке на сутки вперед [800 руб/(МВт ⋅ ч)] инвестиции в АФП и дополнительную турбину не окупаются. При увеличении разницы в ценах (средних за расчетный период 25 лет) на электроэнергию в пиковый и ночной периоды до 3400 руб/(МВт ⋅ ч) окупаемость инвестиций обеспечивается только при разрядке АФП и работе дополнительной паровой турбины более 12 ч в сутки.
Для практического внедрения АФП в тепловую схему АЭС, безусловно, потребуются доработки на уровне проектной организации. К ним можно отнести выбор места размещения АФП на территории АЭС, оценку гидравлических режимов и затрат на перекачку части питательной воды, направляемой в АФП, затрат на модернизацию распределительных устройств и всей электросхемы в целом для подключения дополнительного генератора. Все эти аспекты необходимо учитывать на этапе проектирования и модернизации АЭС, ее электросхемы и генерального плана. Данная технология все еще нуждается в дальнейших исследованиях, в том числе экспериментальных, так как результаты, полученные при теоретических расчетах, указывают на целесообразность проведения предпроектных проработок.
Следует отметить, что комбинирование АФП с дополнительной турбиной в тепловой схеме АЭС способствует повышению эффективности атомной электростанции. В аварийных ситуациях с полным обесточиванием АЭС дополнительная ПТУ может быть применена для электроснабжения систем расхолаживания реакторов (при использовании остаточного тепловыделения остановленного реактора), что позволит повысить уровень безопасности АЭС в соответствии с возрастающими требованиями Международного агентства по атомной энергии и отказаться от дорогостоящих дополнительных систем пассивного отвода тепла (СПОТ). В настоящее время в зарубежной практике для повышения безопасности АЭС на энергоблоках с водо-водяными энергетическими реакторами осуществляется установка дополнительных водяных или воздушных систем пассивного отвода тепла, что требует значительных капиталовложений. Так, по данным официального сайта о размещении заказов на закупки товаров, работ и услуг для нужд Госкорпорации “Росатом”, при строительстве одного энергоблока на Курской АЭС-2 (2018 г.) капиталовложения в воздушные теплообменники СПОТ составили 1094 млн руб.
Учет многофункциональных свойств турбины мощностью 12 МВт при отказе от дополнительных СПОТ обеспечивает экономическую эффективность и оправдывает систему аккумулирования даже при существующих тарифах на электроэнергию, отпускаемую в дневные и ночные часы.
ВЫВОДЫ
1. В качестве теплоаккумулирующего материала в схеме установки аккумулятора фазового перехода с подогревом питательной воды после подогревателей высокого давления двухконтурной АЭС было предложено использовать нитрат лития LiNO3. Данный материал имеет более высокую удельную теплоту фазового перехода по сравнению с другими рассмотренными материалами, что позволяет ему запасать необходимое количество энергии при меньших массе ТАМ и размерах АФП.
2. Моделирование нестационарного теплообмена между теплоаккумулирующим материалом и водой для оребренных и неоребренных труб применительно к элементарной секции АФП методом конечных элементов на основе программного комплекса для ЭВМ позволило заключить следующее. Для выбранного расхода воды через ячейку АФП 0.023 кг/с при длине оребренной трубы 2 м часть питательной воды в первую минуту разрядки нагрелась на 14°С (до 234°С), со второй минуты – на 10°С (до 230°С) и в конце первого часа – на 6.6°С (до 226.6°С). Через 1 ч работы ячейки АФП остаточная часть аккумулированного тепла составляла 26.8%.
3. При текущей разнице в ценах на электроэнергию в ночные и пиковые часы на оптовом рынке электроэнергии и мощности на сутки вперед [800 руб/(МВт ⋅ ч)] инвестиции в АФП и дополнительную турбину не окупаются. При увеличении разницы в ценах на электроэнергию (средних за расчетный период 25 лет) в пиковый и ночной периоды до 3400 руб/(МВт ⋅ ч) окупаемость инвестиций возможна только при разрядке АФП и работе дополнительной паровой турбины более 12 ч в сутки. Учет многофункциональных свойств дополнительной турбины (резервирование собственных нужд АЭС) при отказе от систем пассивного отвода тепла обеспечивает экономическую эффективность и оправдывает систему аккумулирования уже при существующих тарифах на электроэнергию, вырабатываемую в часы как спада, так и пика нагрузки.
Список литературы
Thermo-economic assessment of flexible nuclear power plants in future low-carbon electricity systems: Role of thermal energy storage / A.A. Al Kindi, M. Aunedi, A.M. Pantaleo, G. Strbac, C.N. Markides // Energy Convers. Manage. 2022. V. 258. P. 115484. https://doi.org/10.1016/j.enconman.2022.115484
Carlson F., Davidson J.H. Parametric study of thermodynamic and cost performance of thermal energy storage coupled with nuclear power // Energy Convers. Manage. 2021. V. 236. P. 114054. https://doi.org/10.1016/j.enconman.2021.114054
Чаховский В.М. Аккумуляторы тепла на АЭС // Атомная энергия. 1984. Т. 56. Вып. 6. С. 389–395.
Проект первой маневренной АЭС с аккумуляторами тепла / А.Е. Власов, М.Е. Воронков, М.З. Кривошей, Ю.А. Мостовой, В.М. Чаховский // Атомная энергия. 1987. Т. 62. Вып. 5. С. 302–307.
Боровков В.М., Кушаков А.В. Повышение маневренности АЭС с помощью аккумуляторов энергии // Изв. вузов. Проблемы энергетики. 2010. № 5–6. С. 135–139.
Бажанов В.В., Лощаков И.И., Щуклинов А.П. Исследование возможности использования на АЭС аккумуляторов тепловой энергии при регулировании частоты тока в сети // Изв. вузов. Ядерная энергетика. 2013. № 4. С. 29–36.
Аминов Р.З. Применение многофункциональных систем с тепловыми аккумуляторами фазового перехода как путь повышения безопасности и эффективности АЭС // Теплоэнергетика. 2022. № 8. С. 5–13. https://doi.org/10.56304/S004036362208001X
Aminov R., Yurin V., Murtazov M. Efficiency and economic assessment of combining nuclear power plants with multifunctional heat accumulation systems // Int. J. Energy Res. 2021. V. 45. Is. 8. P. 12464–12473. https://doi.org/10.1002/er.6580
Аминов Р.З., Гариевский М.В. Оценка эффективности АЭС при использовании аккумуляторов фазового перехода // Теплоэнергетика. 2023. № 2. С. 78–89. https://doi.org/10.56304/S0040363623020017
Абросимова Н.И., Каверзнев М.М. К вопросу о конкурентоспособности системы аккумулирования тепловой энергии как способа организации маневренного режима АЭС // Вестник МЭИ. 2016. № 5. С. 57–62.
Rocha T.T.M., Trevizoli P.V., de Oliveira R.N. A timeline of the phase-change problem for latent thermal energy storage systems: A review of theoretical approaches from the 1970′s to 2022 // Sol. Energy. 2023. V. 250. P. 248–284. https://doi.org/10.1016/j.solener.2022.12.035
Liu B., Zhang X., Ji J. Review on solar collector systems integrated with phase-change material thermal storage technology and their residential applications // Int. J. Energy Res. 2021. V. 45. Is. 6. C. 8347–8369. https://doi.org/10.1002/er.6397
A review on current status and challenges of inorganic phase change materials for thermal energy storage systems / S.A. Mohamed, F.A. Al-Sulaiman, N.I. Ibrahim, Md. H. Zahir, A. Al-Ahmed, R. Saidur, B.S. Yılbaş, A.Z. Sahin // Renewable Sustainable Energy Rev. 2017. V. 70. P. 1072–1089. https://doi.org/10.1016/j.rser.2016.12.012
Nomura T., Okinaka N., Akiyama T. Technology of latent heat storage for high temperature application: A review // ISIJ Intern. 2010. V. 50. Is. 9. P. 1229–1239. https://doi.org/10.2355/isijinternational.50.1229
Latent heat thermal energy storage systems with solid-liquid phase change materials: A review / N. Zhang, Y. Yuan, X. Cao, Y. Du, Z. Zhang, Y. Gui // Adv. Eng. Mater. 2018. V. 20. Is. 6. P. 1700753. https://doi.org/10.1002/adem.201700753
Бекман Г., Гилли П. Тепловое аккумулирование энергии: пер. с англ. М.: Мир, 1987.
Dinçer İ., Rosen M.A. Thermal energy storage: systems and applications. John Wiley & Sons, 2010.
A review on phase-change materials: Mathematical modeling and simulations / Y. Dutil, D.R. Rousse, N.B. Salah, S. Lassue, L. Zalewski // Renewable Sustainable Energy Rev. 2011. V. 15. Is. 1. P. 112–130. https://doi.org/10.1016/j.rser.2010.06.011
Development of high temperature phase-change-material storages / D. Laing, T. Bauer, N. Breidenbach, B. Hachmann, M. Johnson // Appl. Energy. 2013. V. 109. P. 497–504. https://doi.org/10.1016/j.apenergy.2012.11.063
High temperature latent heat thermal energy storage integration in a co-gen plant / M. Johnson, J. Vogel, M. Hempel, A. Dengel, M. Seitz, B. Hachmann // Energy Procedia. 2015. V. 73. P. 281–288. https://doi.org/10.1016/j.egypro.2015.07.689
Бабаев Б.Д. Принципы теплового аккумулирования и используемые теплоаккумулирующие материалы // ТВТ. 2014. Т. 52. № 5. С. 760–776. https://doi.org/10.7868/S0040364414050019
A ranking methodology for the coupling of pressurized water nuclear reactors and molten salt thermal energy storage / J. Wallace, C.J. Hirschi, C. Vann, M. Memmott // J. Energy Storage. 2023. V. 59. P. 106562. https://doi.org/10.1016/j.est.2022.106562
Selection principles and thermophysical properties of high temperature phase change materials for thermal energy storage: A review / G. Wei, G. Wang, C. Xu, X. Ju, L. Xing, X. Du, Y. Yang // Renewable Sustainable Energy Rev. 2018. V. 81. P. 1771–1786. https://doi.org/10.1016/j.rser.2017.05.271
Khan M.I., Asfand F., Al-Ghamdi S.G. Progress in research and development of phase change materials for thermal energy storage in concentrated solar power // Appl. Therm. Eng. 2023. V. 219. Part B. P. 119546. https://doi.org/10.1016/j.applthermaleng.2022.119546
Zhou C., Wu S. Medium- and high-temperature latent heat thermal energy storage: Material database, system review, and corrosivity assessment // Int. J. Energy Res. 2019. V. 43. Is. 2. P. 621–661. https://doi.org/10.1002/er.4216
Jankowski N.R., McCluskey F.P. A review of phase change materials for vehicle component thermal buffering // Appl. Energy. 2014. V. 113. P. 1525–1561. https://doi.org/10.1016/j.apenergy.2013.08.026
Kenisarin M.M. High-temperature phase change materials for thermal energy storage // Renewable Sustainable Energy Rev. 2010. V. 14. Is. 3. P. 955–970. https://doi.org/10.1016/j.rser.2009.11.011
Investigation of latent heat thermal energy storage materials: V. thermoanalytical evaluation of binary eutectic mixtures and compounds of NAOH with NaNO3 or NaNO2 / Y. Takahashi, M. Kamimoto, Y. Abe, R. Sakamoto, K. Kanari, T. Ozawa // Thermochim. Acta. 1988. V. 123. P. 233–245. https://doi.org/10.1016/0040-6031(88)80027-3
Latent thermal energy storage for solar process heat applications at medium-high temperatures – A review / A. Crespo, C. Barreneche, M. Ibarra, W. Platzer // Sol. Energy. 2019. V. 192. P. 3–34. https://doi.org/10.1016/j.solener.2018.06.101
Latent heat storage above 120°C for applications in the industrial process heat sector and solar power generation / R. Tamme, T. Bauer, J. Buschle, D. Laing, H. Müller-Steinhagen, W.-D. Steinmann // Int. J. Energy Res. 2008. V. 32. Is. 3. C. 264–271. https://doi.org/10.1002/er.1346
Thermophysical, environmental, and compatibility properties of nitrate and nitrite containing molten salts for medium temperature CSP applications: A critical review / T. Delise, A.C. Tizzoni, M. Ferrara, N. Corsaro, C. D’Ottavi, S. Sau, S. Licoccia // J. European Ceramic Soc. 2019. V. 39. Is. 1. P. 92–99. https://doi.org/10.1016/j.jeurceramsoc.2018.07.057
Review of thermal materials for CSP plants and LCOE evaluation for performance improvement using Chilean strategic minerals: lithium salts and copper foams / G. Cáceres, M. Montané, S. Nasirov, R. O’Ryan // Sustainability. 2016. V. 8. Is. 2. P. 106. https://doi.org/10.3390/su8020106
Molten salt latent thermal energy storage for load following generation in nuclear power plants / Y. Abe, Y. Takahashi, K. Kanari, M. Kamimoto, R. Sakamoto, T. Ozawa // Proc. of the 21st Intersociety Energy Conversion Engineering Conf. San Diego, California, USA, 25–29 Aug. 1986. P. 856–861.
Thermal conductivity enhancement of phase change materials for thermal energy storage: A review / L. Liu, D. Su, Y. Tang, G. Fang // Renewable Sustainable Energy Rev. 2016. V. 62. P. 305–317. https://doi.org/10.1016/j.rser.2016.04.057
Hariss M., Gounni A., El Alami M. A practical guide on numerical and experimental investigations of solid-liquid phase-change heat transfer using some heat transfer enhancement techniques: Case study in Morocco // J. Energy Storage. 2022. V. 52. Part C. P. 105070. https://doi.org/10.1016/j.est.2022.105070
Highly conductive composites made of phase change materials and graphite for thermal storage / S. Pincemin, R. Olives, X. Py, M. Christ // Sol. Energy Mater. Sol. Cells. 2008. V. 92. Is. 6. P. 603–613. https://doi.org/10.1016/j.solmat.2007.11.010
Шишкин Н.Д., Цымбалюк Ю.В. Фазопереходные тепловые аккумуляторы с высокотеплопроводными инклюзивами: монография. Астрахань: Новая Линия, 2006.
Dhaidan N.S., Khodadadi J.M. Improved performance of latent heat energy storage systems utilizing high thermal conductivity fins: A review // J. Renewable Sustainable Energy. 2017. V. 9. Is. 3. P. 034103. https://doi.org/10.1063/1.4989738
Performance evaluation of latent heat TES system-case study: Dimensions improvements of annular fins exchanger / Z. Elmaazouzi, M. El Alami, H. Agalit, E.G. Bennouna // Energy Rep. 2020. V. 6. P. 294–301. https://doi.org/10.1016/j.egyr.2019.08.059
Vogel J., Keller M., Johnson M. Numerical modeling of large-scale finned tube latent thermal energy storage systems // J. Energy Storage. 2020. V. 29. P. 101389. https://doi.org/10.1016/j.est.2020.101389
Effect of shell shape on the charging and discharging performance of a vertical latent heat thermal energy storage unit: An experimental study / B. Lu, Y. Zhang, Z. Wang, J. Zhu, J. Zhang, D. Sun // J. Energy Storage. 2022. V. 56. Part A. P. 105996. https://doi.org/10.1016/j.est.2022.105996
Heat transfer enhancement of phase change materials by fins under simultaneous charging and discharging / M.M. Joybari, F. Haghighat, S. Seddegh, A.A. Al-Abidi // Energy Convers. Manage. 2017. V. 152. P. 136–156. https://doi.org/10.1016/j.enconman.2017.09.018
Vogel J., Johnson M. Natural convection during melting in vertical finned tube latent thermal energy storage systems // Appl. Energy. 2019. V. 246. P. 38–52. https://doi.org/10.1016/j.apenergy.2019.04.011
Vogel J., Thess A. Validation of a numerical model with a benchmark experiment for melting governed by natural convection in latent thermal energy storage // Appl. Therm. Eng. 2019. V. 148. P. 147–159. https://doi.org/10.1016/j.applthermaleng.2018.11.032
Numerical study of the heat charging and discharging characteristics of a shell-and-tube phase change heat storage unit / W.-W. Wang, K. Zhang, L.-B. Wang, Y.-L. He // Appl. Therm. Eng. 2013. V. 58. Is. 1–2. P. 542–553. https://doi.org/10.1016/j.applthermaleng.2013.04.063
Ali M., Alkaabi A.K., Lee J.I. CFD simulation of an integrated PCM-based thermal energy storage within a nuclear power plant connected to a grid with constant or variable power demand // Nucl. Eng. Des. 2022. V. 394. P. 111819. https://doi.org/10.1016/j.nucengdes.2022.111819
Sizing the thermal energy storage device utilizing phase change material (PCM) for low-temperature organic Rankine cycle systems employing selected hydrocarbons / S. Daniarta, M. Nemś, P. Kolasiński, M. Pomorski // Energies. 2022. V. 15. Is. 3. P. 956. https://doi.org/10.3390/en15030956
Experimental and numerical analysis of a phase change material-based shell-and-tube heat exchanger for cold thermal energy storage / A. Fragnito, N. Bianco, M. Iasiello, G.M. Mauro, L. Mongibello // J. Energy Storage. 2022. V. 56. P. 105975. https://doi.org/10.1016/j.est.2022.105975
Sensitivity analysis and optimization of structural parameters of a phase change material based multi-tube heat exchanger under charging condition / X. Ma, Q. Zhang, J. Wang, C. Yue // J. Energy Storage. 2022. V. 56. Part B. P. 105940. https://doi.org/10.1016/j.est.2022.105940
Пат. 2680380 РФ, МПК G21D 5/00. Способ повышения мощности и безопасности энергоблока АЭС с реактором типа ВВЭР на основе теплового аккумулирования / Р.З. Аминов, В.Е. Юрин, М.А. Муртазов. Заявители и патентообладатели Р.З. Аминов, В.Е. Юрин, М.А. Муртазов. Заявл. 01.12.2017. Опубл. 20.02.2019 // Б. И. 2019. № 5.
Дополнительные материалы отсутствуют.
Инструменты
Теплоэнергетика