Радиационная биология. Радиоэкология, 2023, T. 63, № 4, стр. 394-402
Метод ДНК-комет не выявил снижения повреждений ДНК лимфоцитов, вызванных рентгеновским излучением, при действии натрий-медного хлорофиллина в концентрации до 100 мкмоль/л
Л. А. Ромодин 1, *, М. А. Игнатов 1
1 Государственный научный центр Российской Федерации – Федеральный медицинский биофизический центр
им. А.И. Бурназяна ФМБА России
Москва, Россия
* E-mail: fmbc@fmbamail.ru
Поступила в редакцию 17.01.2023
После доработки 22.05.2023
Принята к публикации 21.06.2023
- EDN: EHTQMJ
- DOI: 10.31857/S0869803123040070
Аннотация
Поиск эффективных, но не токсичных радиозащитных средств остается главной задачей радиобиологии. По ряду сообщений к таковым могут относиться препараты на основе хлорофилла, в частности, хлорофиллина – водорастворимого продукта его омыления. Так как многие исследователи отводят повреждениям ДНК ключевую роль в развитии негативных последствий действия ионизирующего излучения, было проведено настоящее исследование по воздействию рентгеновского излучения суспензии лимфоцитов в растворах натрий-медного хлорофиллина диапазоне концентраций 5–100 мкмоль/л. Щелочная модификация метода гель-электрофореза отдельных клеток не показала достоверных отличий в содержании ДНК в хвосте и моменте хвоста ДНК-комет облученных лимфоцитов, инкубированных в хлорофиллине, по сравнению с только облученными клетками. Данный результат можно объяснить тем, что, скорее всего, хлорофиллин не проходит через ядерную мембрану из цитоплазмы в ядро. И именно отсутствием хлорофиллина в ядре можно объяснить то, что степень повреждения ДНК в опытных пробах не отличалась от таковой в контрольной.
Ввиду высокой химической токсичности всех применяемых в настоящее время радиопротекторов [1], крайне актуальной остается задача поиска нетоксичных, но при том эффективных радиозащитных средств. И хотя данную проблему пытаются решить на протяжении длительного времени [2–11], считать ее решенной на настоящий момент не приходится [12].
Однако в литературе имеются сведения о том, что препараты на основе хлорофилла, обладающие очень низкой токсичностью, проявляют радиозащитный эффект [13–18]. В некоторых из данных работ изучались радиопротекторные свойства непосредственно самого хлорофилла [13–15], в других – хлорофиллина – водорастворимого продукта его омыления [16–18].
Недавно методом регистрации усиленной хинолизидин[5,6,7-gh]3-ацетилкумарином (coumarin-334) хемилюминесценции, сопровождающей катализируемую комплексом цитохрома c с кардиолипином квазилипоксигеназную реакцию, показано подавление хлорофиллином запущенного данным процессом перекисного окисления липидов [19]. Данный результат позволяет предположить, что в основе радиозащитного действия препаратов на основе хлорофилла может лежать подавление перекисного окисления липидов. Но при этом факт подавления препаратами на основе хлорофилла липидной пероксидации вовсе не отменяет их возможных генопротекторных свойств – гипотетической способности защищать молекулы ДНК от радиационных поражений. Главным механизмом действия данных поражений является атака на них продуктов радиолиза воды – гидроксильных и гидропероксильных радикалов [20].
Так как многие исследователи основополагающую роль в патогенезе лучевого поражения отводят именно повреждениям ДНК [21–24], мы провели эксперимент по оценке способности хлорофиллина предотвращать повреждения ДНК у лимфоцитов, подвергнутых действию рентгеновского облучения.
Данная оценка была проведена методом гель-электрофореза одиночных клеток. Данный метод заключается в смешении клеточной суспензии с агарозным гелем с последующим лизированием клеточных структур, за исключением ДНК, и помещении образцов в электрическое поле. В нем ДНК, будучи отрицательно заряженной молекулой , “пытается” двигаться в сторону анода. Однако структура агарозы затрудняет это движение, поэтому в толще геля с бо́льшим успехом проходят небольшие фрагменты ДНК, имеющие место в образце вследствие повреждения молекул ДНК. Поэтому образуется кометаподобная структура, на основании выраженности хвоста в которой можно судить о степени разрушения ДНК под действием различных факторов, влияниям которых подвергалась клетка [25–27]. Подобным образом можно изучать двухнитевые разрывы ДНК.
Однако, если проводить электрофорез в щелочной среде, становится возможным детектировать и однонитевые разрывы ДНК, а также так называемые щелочно-лабильные сайты – разрывающиеся в щелочной среде участки ДНК, неустойчивость которых возникает по причине действия поражающего фактора [25, 28], например, рентгеновского излучения.
Если говорить о последних работах с применением метода ДНК-комет, то здесь можно указать работу C.R. Cooper и соавт. [29], в которой при использовании метода ДНК-комет изучены повреждения ДНК, вызванные сверхвысокомощным импульсным FLASH-облучением, и сделан вывод о том, что временное снижение содержания кислорода в тканях – это причина относительно малых деструктивных последствий данного режима облучения. Метод ДНК-комет лег в основу нового метода оценки целостности генома тестикулярных зародышевых клеток [30] и выявления повреждений ДНК для детекции злокачественной трансформации клеток при хроническом циррозе печени [31]. С помощью метода ДНК-комет обнаружены повреждения генетического аппарата лейкоцитов периферической крови у пациентов, страдающих хронической почечной недостаточностью [32].
M. Unverricht-Yeboah и соавт. [33] показали более интенсивное образование двунитевых разрывов ДНК, вызванных включением в ДНК 125I по сравнению с действием γ-излучения с низкой линейной передачей энергии.
Кроме того, метод ДНК-комет может применяться и в экологических исследованиях, например, с его помощью изучали степень загрязненности водоемов, анализируя на предмет генетических повреждений клетки из гемолимфы живущих в них крабов Aegla platensis [34] и степень загрязненности воздуха на основании анализа клеток Бирючины обыкновенной (Ligustrum vulgare) [35].
Большой интерес вызывает работа M. Karbaschi и соавт. [36], разработавших установку для автоматизированного применения метода ДНК-комет. Подобное устройство позволит снизить субъективизм при оценке результатов, в высокой степени свойственный данному методу [37, 38].
Целью же настоящей работы стало проведение исследования возможности натрий-медного хлорофиллина предотвращать возникновение повреждений ДНК в лимфоцитах, суспензия которых подверглась внешнему воздействию рентгеновским излучением.
МАТЕРИАЛЫ И МЕТОДИКА
Выделение лимфоцитов крови. Суспензия изолированных лимфоцитов была приготовлена из жидкой соединительной ткани человека – крови. Лимфоциты выделяли из проб крови пациентов ФГБУ ГНЦ ФМБЦ им. А.И. Бурназяна ФМБА России методом центрифугирования в градиенте плотности. Между забором крови и выделением клеток, а также между выделением клеток и анализом сохранялась температура 4°С. Гепаринизированную кровь развели фосфатно-солевым буфером (РВS), приготовленном путем растворения таблетки (Thermo Fisher Scientific, США) в деионизированной воде, в соотношении 1: 1. Далее в центрифужные пробирки добавили 3 мл раствора фиколла плотностью 1.077 г/мл (ПанЭко, Россия), после чего наслоили на него по 6 мл разведенной крови, избегая перемешивания с ним. Далее пробы центрифугировали при 400 g в течение 30 мин. Далее отбирали опалесцирующее кольцо, содержащее мононуклеары, в чистые центрифужные пробирки, добавив в них по 10 мл PBS, перемешав суспензию. Пробы центрифугировали при 300 g в течение 10 мин, после чего, слив супернатант, ресуспендировали осадок в добавленных 5 мл PBS. Далее снова центрифугировали пробы при 300 g в течение 10 мин и, слив супернатант, ресуспендировали осадок в добавленных 5 мл РBS. После чего еще раз центрифугировали пробы при 300 g в течение 10 мин с последующим ресуспендированием осадка в 1 мл среды RPMI 1640 (полн.). Среда была приготовлена путем добавления к 500 мл среды RPMI Medium 1640 1x (GIBCO (Life Technologies (Invitrogen)), США) 50 мл инактивированной Fetal bovine serum (Thermo Fisher Scientific, США), 146 мг L-глутамина (ПанЭко, РФ), смеси 25 000 ед. пенициллина и 25 мг стрептомицина (ПанЭко, РФ).
Инкубирование лимфоцитов в хлорофиллине и проведение облучения. Суспензию лимфоцитов смешивали в соотношении 1:1 с растворами медного хлорофиллина натрия (CZY prospertity Store, Китай) в среде RPMI 1640 (полн.) следующих концентраций: 10, 50, 100 и 200 мкмоль/л. Контрольные пробы разбавлялись в 2 раза средой RPMI 1640 (полн.), не содержащей хлорофиллин. Далее полученные таким образом суспензии лимфоцитов в течение 1 ч инкубировали в воздушном термостате MCO-15AC фирмы “Sanyo” (Япония) при температуре 37°C. После инкубации половина проб была подвержена воздействию рентгеновского излучения на рентгеновской установке РУСТ-М1, РФ в дозе 2 Гр.
Проведение гель-электрофореза одиночных клеток. Суспензии лимфоцитов после облучения сразу смешивали с раствором легкоплавкой агарозы (Helicon, РФ, 1%), имеющим температуру ≈38°C. Каждая проба состояла из 200 мкл легкоплавкой агарозы и 200 мкл суспензии лимфоцитов с примерной концентрацией ≈106 клеток/мл. Далее 75 мкл получившейся смеси наносили на предметные стекла со слоем тугоплавкой агарозы (Helicon, РФ, 1%) и сразу накрывали покровным стеклом. После чего инкубировали пробы в течение 10 мин при 4°C. Далее покровные стекла снимали и помещали образцы в лизирующий буфер, в котором инкубировали пробы в течение 1 ч при 4°C. При приготовлении лизирующего раствора добавляли 5 мл ДМСО (ПанЭко, РФ) и 0.5 мл Triton X-100 (Helicon, РФ) к 45 мл стокового лизирующего буфера (ХимМед, РФ, состав: NaOH 1%, NaCl 14%, ЭДТА 2%, трис-HCl 0.2%).
Электрофорез проводили в камере (Helicon, РФ) в среде буфера, приготовленного путем смешивания 15 мл 10 н NaOH 15 мл, 10 мл 100 ммоль/л ЭДТА (pH = 10.0) с дистиллированной водой так, чтобы общий объем буфера составлял 500 мл. Перед проведением электрофореза стекла с образцами выдерживали в среде данного буфера в течение 20 мин при температуре 4°C. После этого стекла помещали в электрофоретическую камеру и заливали ее буфером так, чтобы толщина его слоя над стеклами составляла 2–3 мм. Электрофорез проводили в течение 20 мин при напряжении в камере 20 В. Температура буфера составляла 4°C и поддерживалась аккумулятором холода.
После щелочного электрофореза стекла обрабатывали 3 раза по 5 мин нейтрализующим трис-борат-ЭДТА-буфером х10, разведенным в 5 раз, в течение 5 мин. Затем мы выдерживали стекла с образцами в течение 5 мин в дистиллированной воде, после чего – в течение 5 мин в 70%-ном этаноле (приготовлен разведением в деионизированной воде 95%-ного этанола производства АО “РФК”, РФ). Далее стекла с образцами высушивали на фильтровальной бумаге агарозой вверх.
Проведение анализа повреждений ДНК методом ДНК-комет. На стекло с образцом равномерно наносили 20 мкл раствора акридинового оранжевого (0.5%, ПанЭко, РФ), после чего накрывали его покровным стеклом. Далее при использовании флуоресцентного микроскопа “Nikon Eclipse Ni-U” (Nikon, Япония), подключенного к персонального компьютеру, оснащенному программой ProgRes Capture pro, было проведено фотографирование клеток таким образом, чтобы каждой пробе соответствовало 100 клеток. При этом каждой пробе соответствовало два стекла, с каждого из которых анализировали по 50 клеток. Анализ ДНК-комет проводили с использованием программы Casp. В качестве анализируемых параметров использовали процентное содержание ДНК в хвосте ДНК-кометы и момент хвоста – произведение длины кометы на содержание ДНК в ее хвосте [39].
Статистическую обработку результатов проводили с использованием программы Microsoft Office Excel 2016. Данные на графиках представлены в виде: среднее арифметическое с границами доверительного интервала, который был вычислен с использованием t-критерия Стьюдента при доверительной вероятности 95%. Нормальность распределения результатов измерений для каждой экспериментальной точки и контролей была проверена с использованием критерия согласия Пирсона [40].
РЕЗУЛЬТАТЫ
Степень повреждения ДНК лимфоцитов оценивали по двум показателям: проценту содержания ДНК в хвосте ДНК-кометы и моменту хвоста – произведению доли (не процента) содержания ДНК в хвосте на длину хвоста ДНК-кометы. Так как программа Casp обрабатывает фотографии поля зрения микроскопа без учета реальных линейных размеров ДНК-кометы, применяя собственные, условные, единицы длины, полученная величина момента хвоста также измеряется в относительных единицах. Результаты эксперимента проиллюстрированы рис. 1.
Рис. 1.
Показатели степени разрушения ДНК лимфоцитов, инкубированных в среде, содержащей натрий-медный хлорофиллин, подвергнутых после инкубации действию рентгеновского излучения в дозе 2 Гр (треугольные маркеры) и не подвергавшихся облучению (круглые маркеры): а) % ДНК в хвосте ДНК-комет; б) момент хвоста ДНК-комет.
Fig. 1. Indicators of the degree of destruction of DNA of lymphocytes incubated in a medium containing sodium-copper chlorophyllin, exposed after incubation to X-ray radiation at a dose of 2 Gy (triangular markers) and not exposed to radiation (round markers). A. The percentage of DNA in the tail of DNA-comets. B. The tail moment of DNA-comets.
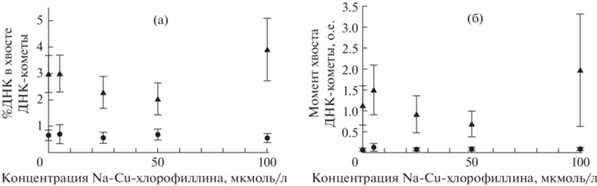
Контрольным пробам на графиках на рис. 1 соответствуют точки, лежащие на оси ординат. Положительный контроль – проба, подвергнутая облучению в дозе 2 Гр без предварительной инкубации в растворе хлорофиллина. На графике ей соответствует треугольный маркер на оси ординат. Отрицательный контроль – лимфоциты, не инкубированные в хлорофиллине и не подвергнутые облучению. На графике – это круглый маркер на оси ординат. Собственно, экспериментальные пробы – это облученные пробы, предварительно инкубированные в растворах хлорофиллина различных концентраций (треугольные маркеры на графиках, за исключением лежащего на оси ординат). Пробы, инкубированные в хлорофиллине без последующего облучения (круглые маркеры на графиках, за исключением лежащего на оси ординат), выступили в роли контролей на действие рентгеновского излучения. Данные измерения призваны были охарактеризовать потенциальное влияние хлорофиллина на степень целостности ДНК лимфоцитов (табл. 1).
Таблица 1.
Показатели степени разрушения ДНК лимфоцитов, инкубированных в среде, содержащей натрий-медный хлорофиллин, подвергнутых после инкубации действию рентгеновского излучения в дозе 2 Гр Table 1. Indicators of the degree of destruction of DNA of lymphocytes incubated in a medium containing sodium-copper chlorophyllin, exposed after incubation to X-ray radiation at a dose of 2 Gy
Концентрация хлорофиллина, мкмоль/л | Доза рентгеновского излучения, Гр | % ДНК в хвосте ДНК-кометы | Момент хвоста ДНК-кометы | Тип пробы |
---|---|---|---|---|
0 | 0 | 0.64 ± 0.20 | 0.07 ± 0.03 | отрицательный контроль |
5 | 0 | 0.69 ± 0.37 | 0.12 ± 0.10 | контроль на радиацию |
25 | 0 | 0.55 ± 0.23 | 0.07 ± 0.03 | контроль на радиацию |
50 | 0 | 0.67 ± 0.21 | 0.10 ± 0.04 | контроль на радиацию |
100 | 0 | 0.56 ± 0.13 | 0.08 ± 0.03 | контроль на радиацию |
0 | 2 | 2.96 ± 0.71 | 1.12 ± 0.47 | положительный контроль |
5 | 2 | 2.97 ± 0.70 | 1.49 ± 0.59 | опытная проба |
25 | 2 | 2.25 ± 0.61 | 0.91 ± 0.45 | опытная проба |
50 | 2 | 2.06 ± 0.60 | 0.69 ± 0.30 | опытная проба |
100 | 2 | 3.93 ± 1.21 | 1.96 ± 1.36 | опытная проба |
Между пробами, получившими 2 Гр рентгеновского излучения, и необлученными пробами наблюдаются достоверные отличия по обоим параметрам. Однако внутри групп как облученных, так и необлученных проб достоверных отличий степени повреждения ДНК между образцами, инкубированных в растворах хлорофиллина различных концентраций и не инкубированных в нем, не обнаружено.
ОБСУЖДЕНИЕ
Согласно данным, представленным на рис. 1, медный хлорофиллин в нашем исследовании как не показал генопротекторного эффекта, так и не продемонстрировал роли радиосенсибилизатора – вещества, усиливающего повреждающее действие радиации.
Учитывая результаты исследований [16, 17, 19, 41], показавших радиозащитные свойства хлорофиллина, можно предложить следующие варианты ответа на вопрос, почему наш эксперимент дал отрицательный результат. Во-первых, хлорофиллин мог не проникать в ядра лимфоцитов или сами лимфоциты, а потому не вызвал угнетения метаболизма активных форм кислорода (АФК) в окружении ДНК. Это предположение логично потому, что водорастворимая молекула хлорофиллина должна крайне плохо диффундировать в липидном бислое мембраны. Во-вторых, примененные концентрации хлорофиллина могли быть недостаточными для эффективного подавления активности радиогенных АФК. Или же в клетки из среды поступило недостаточно хлорофиллина для эффективного подавления деструктивных процессов, вызванных действием рентгеновского излучения: при, казалось бы, достаточной концентрации хлорофиллина в среде внутриклеточная и, тем более, внутриядерная концентрация, оказались слишком малы для ингибирования метаболизма АФК.
В качестве аргументов в пользу необходимости использования более высоких концентраций хлорофиллина выступает то, что в работах [16, 17, 41] и некоторых опытах, проведенных S.S. Kumar и соавт. [18], положительные результаты наблюдались при использовании хлорофиллина в концентрациях, превышающих 100 мкмоль/л. Однако в этих концентрациях хлорофиллин оказывал радиозащитное действие на генетический аппарат: у облученных мышей под действием хлорофиллина уменьшалось число микроядерных полихроматических эритроцитов [41] и снижалась вероятность сестринских хроматидных обменов в клетках костного мозга [17] и спермогониях [16]. К слову, выводы первой работы, посвященной изучению радиозащитных свойств хлорофиллина, объявляют о способности хлорофиллина защищать генетический аппарат при действии ионизирующего излучения [42]. Тогда благодаря хлорофиллину на крыльях облученных мушек Drosophila melanogaster снизилось количество пятен – маркеров генетических повреждений.
Используемые же нами в настоящей работе концентрации хлорофиллина были выбраны на основании результатов, описанных в статье [19]. Тогда при концентрациях хлорофиллина, начиная от 1.56 мкмоль/л, наблюдалось достоверное подавление интенсивности липидной пероксидации, запущенной квазилипоксигеназной реакцией, катализируемой комплексом цитохрома c с кардиолипином. Здесь можно заметить, что в работе [19] экспериментальная модельная система представляла собой раствор, а в настоящем исследовании – клетки, пусть и в виде суспензии. И поэтому сравнивать их между собой не вполне корректно. Однако S.S. Kumar и соавт. [18] проводили эксперимент, в числе прочего, на суспензии лимфоцитов, облученных γ-излучением. Данную систему можно считать аналогичной нашей, за исключением того, что в исследовании [18] использовали мышиные лимфоциты, а не человеческие, и использовали γ-излучение в дозе 2.5 Гр, а не рентгеновское в дозе 2 Гр.
S.S. Kumar и соавт. [18] по флуоресценции дихлорфлуоресцеина изучали влияние хлорофиллина на метаболизм АФК в облученных лимфоцитах. И хлорофиллин концентрацией уже 0.1 мкмоль/л достоверно подавлял его. Основываясь на этих данных, можно говорить, что и в нашем исследовании, при концентрациях хлорофиллина 5–100 мкмоль/л, уровень радиогенных АФК в лимфоцитах снижался. Но это не снизило число повреждений ДНК.
Говоря о воздействии ионизирующего излучения, необходимо учитывать вклад прямого попадания квантов излучения в биомолекулы и вклад их реакции с продуктами радиолиза воды. Хотя здесь необходимо учитывать отсутствие четкой границы между данными терминами. Так, по эффекту попадание кванта излучения в гидратную оболочку ДНК можно считать прямым попаданием в последнюю [43]. Ясно, что ни один антиоксидант не может спасти ДНК (как и любую другую биомолекулу) от непосредственного попадания в нее кванта рентгеновского излучения.
Ввиду того, что ДНК относительно других структур занимает небольшой объем в клетке, оценка последствий действия радиации по содержанию АФК слабо отражает степень повреждения именно молекул ДНК. Данный способ оценки не учитывает прямое воздействие ионизирующего излучения на ДНК, а отражает интенсивность процессов с участием продуктов радиолиза воды, которые хотя и могут провзаимодействовать с молекулой ДНК, с бо́льшей вероятностью окислят липиды, которых в клетке больше. Расстояние, на которое в ядре клетки может диффундировать гидроксильный радикал до взаимодействия с другой молекулой, по информации из обзора [43], не превышает 1 нм. Но здесь необходимо иметь в виду возможность запуска цепной свободнорадикальной реакции.
Приведенные выше результаты исследования [18] по снижению содержания АФК в облученных клетках под действием хлорофиллина в концентрациях от 0.1 мкмоль/л не вступают в противоречие с полученными нами в настоящей работе данными. Хлорофиллин в облученных клетках подавил метаболизм липидных радиотоксинов, мог непосредственно перехватить часть продуктов радиолиза воды, и все это привело к резкому снижению содержания АФК в клетках. Однако по причине того, что лишь очень малая часть АФК задействована в атаках на ДНК, общее снижение фона АФК в клетках не смогло дать достоверного снижения степени поврежденности ДНК.
Но тут необходимо вспомнить о результатах работы M.J. Peak и соавт. [44], показавших, что вещества, “гасящие” гидроксильные радикалы, защищают ДНК от разрывов, вызванных действием ультрафиолетового и γ-излучения. Однако данное исследование проводилось in vitro: ДНК находилась в растворе, в котором присутствовали восстановители, причем в концентрациях, превышающих таковые, используемые нами для хлорофиллина. Наименьшая концентрация восстановителя в исследовании [45] была равна 650 мкмоль/л – для азида – вещества с настолько большой восстанавливающей способностью (очевидно, бо́льшей, чем у хлорофиллина), что она делает его токсичным для живых клеток [46].
ЗАКЛЮЧЕНИЕ
В свете всего вышесказанного можно предположить, что концентрация хлорофиллина в ядрах лимфоцитов оказалась недостаточной для перехвата АФК, приведших к разрушению их ДНК. Далее остается выяснить, по какой причине. Это может быть обусловлено как тем, что применяемые нами концентрации хлорофиллина (до 100 мкмоль/л) в принципе не способны хоть в какой-нибудь степени препятствовать радиационно-индуцированным повреждениям ДНК, так и тем, что хлорофиллин не проникает или очень плохо проникает из среды в цитоплазму, а из цитоплазмы – в ядро клеток. Поэтому в будущих исследованиях необходимо выяснить, насколько хорошо хлорофиллин может проникать в живые клетки из среды, в которой они находятся.
Список литературы
Иванов И.М., Никифоров А.С., Юдин М.А. и др. Перспективы ингаляционной доставки медицинских средств защиты при радиационных поражениях // Радиац. биология. Радиоэкология. 2020. Т. 60. № 2. С. 175–188. [Ivanov I.M., Nikiforov A.S., Yudin M.A. et al. Prospects for inhalation delivery of medical protectors in radiation damage // Radiation biology. Radioecology. 2020. V. 60. № 2. P. 175–188. (In Russ.)]. https://doi.org/10.31857/S0869803120020058
Naik G.H., Priyadarsini K.I., Naik D.B. et al. Studies on the aqueous extract of Terminalia chebula as a potent antioxidant and a probable radioprotector // Phytomedicine: Int. J. Phytotherap. Phytopharmacol. 2004. V. 11. № 6. P. 530–538. https://doi.org/10.1016/j.phymed.2003.08.001
Metodiewa D., Kochman A., Karolczak S. Evidence for antiradical and antioxidant properties of four biologically active N,N-diethylaminoethyl ethers of flavanone oximes: a comparison with natural polyphenolic flavonoid (rutin) action // Biochem. Mol. Biol. Int. 1997. V. 41. № 5. P. 1067–1075. https://doi.org/10.1080/15216549700202141
Narra V.R., Harapanhalli R.S., Howell R.W. et al. Vitamins as radioprotectors in vivo. I. Protection by vitamin C against internal radionuclides in mouse testes: implications to the mechanism of damage caused by the Auger effect // Radiat. Res. 1994. V. 137. № 3. P. 394–399.
Harapanhalli R.S., Narra V.R., Yaghmai V. et al. Vitamins as radioprotectors in vivo. II. Protection by vitamin A and soybean oil against radiation damage caused by internal radionuclides // Radiat. Res. 1994. V. 139. № 1. P. 115–122.
Liang P.S., Haff R.P., Ovchinnikova I. et al. Curcumin and Quercetin as Potential Radioprotectors and/or Radiosensitizers for X-ray-based Sterilization of Male Navel Orangeworm Larvae // Sci. Rep. 2019. V. 9. № 1. P. 2016. https://doi.org/10.1038/s41598-019-38769-3
Shivappa P., Bernhardt G.V. Natural Radioprotectors on Current and Future Perspectives: A Mini-Review // J. Pharm. & Bioall. Sci. 2022. V. 14. № 2. P. 57–71. https://doi.org/10.4103/jpbs.jpbs_502_21
Томусяк М.В., Соколов И.Р., Горюнов М.А., Колесников А.С. Потенциал применения растений в препаратах-радиопротекторах // Интернаука. 2022. № 11-1. № 234. С. 11–14. [Tomusyak M., Sokolov I., Goryunov M., Kolesnikov A. Potential of application of plants in radioprotective drugs // Internauka. 2022. V. 11–1. № 234. P. 11–14. (In Russ.)]
Софронов Г.А., Мурзина Е.В., Аксенова Н.В. и др. Перспективы изучения эффективности бета-D-глюканов в качестве противолучевых средств // Изв. Рос. воен.-мед. академии. 2020. № 39. № S3-3. С. 193–198. [Sofronov G.A., Murzina E.V., Aksenova N.V. et al. Study perspectives of the effectiveness of beta-D-glucans as an antiradiation agents // Russian Military Medical Academy Reports. 2020. V. 39. № S3–3. P. 193–198. (In Russ.)]
Воронцова З.А., Иванов А.А., Никитюк Д.Б., Аванесова А.А. Некоторые морфоклинические доказательства радиопротективного характера меланинов (обзор литературы) // Вестн. новых медицинских технологий. Электронное издание. 2016. № 4. С. 295–302. [Vorontsova Z.A., Ivanov A.A., Nikityuk D.B. Some morphoclinical evidence about radioprotective melanin character (literature report) // Journal of New Medical Technologies, eEdition. 2016. V. 4. P. 295–302. (In Russ.)]. https://doi.org/10.12737/22219
Попова Н.Р., Гудков С.В., Брусков В.И. Природные пуриновые соединения как радиозащитные средства // Радиац. биология. Радиоэкология. 2014. Т. 54. № 1. С. 38–49. [Popova N.R., Gudkov S.V., Bruskov V.I. Natural Purine Compounds as Radioprotective Agents // Radiacionnaya biologiya. Radio-ekologiya. 2014. V. 54. № 1. P. 38–49. (In Russ.)]. https://doi.org/10.7868/S0869803114010135
Рождественский Л.М. Проблемы разработки отечественных противолучевых средств в кризисный период: Поиск актуальных направлений развития // Радиац. биология. Радиоэкология. 2020. Т. 60. № 3. С. 279–290. [Rozhdestvensky L.M. Difficulties in radiation counter measure preparations deve-lopment in russiain crysis period: actual approaches searchin // Radiacionnaya biologiya. Radioekologiya. 2020. V. 60. № 3. P. 279–290. (In Russ.)]. https://doi.org/10.31857/S086980312003011X
Поздеев А.В., Гугало В.П. Влияние препарата хлорофилла на содержание малонового диальдегида при радиационной патологии // Вестн. Курской гос. сельскохозяйственной академии. 2012. № 2. С. 107–109. [Pozdeev A.V., Gugalo V.P. Vliyanie preparata hlorofilla na soderzhanie malonovogo dial’degida pri radiacionnoj patologii // Vestnik Kurskoj gosudarstvennoj sel’skohozyajstvennoj akademii. 2012. V. 2. P. 107–109. (In Russ.)]
Поздеев А.В., Лысенко Н.П. Повышение радиационной устойчивости организма млекопитающих при применении препаратов хлорофилла в условиях радиоактивного загрязнения окружающей среды // Изв. Междунар. академии аграрного образования. 2018. Вып. 42. Т. 2. С. 60–62. [Pozdeev A.V., Lysenko N.P. increase in radiation stability of an orga-nism mammals at use of medicines of a chlorophyll in the conditions of radioactive environmental pollution // Izvestiya Mezhdunarodnoj akademii agrarnogo obrazovaniya. 2018. V. 42. № 2. P. 60–62. (In Russ.)]
Поздеев А.В., Промоненков В.К., Лысенко Н.П. Применение растительного пигмента в качестве ингибитора электронно-возбужденных состояний // Вет. медицина. 2010. № 2010–1. С. 42–43. [Pozdeev A.V., Promonenkov V.K., Lysenko N.P. Application of the vege-tative pigment in quality retarding agent at set states of electron // Veterinarnaya medicina. 2010. V. 2010-1. P. 42–43. (In Russ.)]
Morales-Ramirez P., Mendiola-Cruz M.T. In vivo radioprotective effect of chlorophyllin on sister chromatid exchange induction in murine spermatogonial cells // Mutat. Res. 1995. V. 344. № 1–2. P. 73–78. https://doi.org/10.1016/0165-1218(95)90041-1
Morales-Ramirez P., Garcia-Rodriguez M.C. In vivo effect of chlorophyllin on gamma-ray-induced sister chromatid exchange in murine bone marrow cells // Mutat. Res. 1994. V. 320. № 4. P. 329–334. https://doi.org/10.1016/0165-1218(94)90085-x
Kumar S.S., Shankar B., Sainis K.B. Effect of chlorophyllin against oxidative stress in splenic lymphocytes in vitro and in vivo // Biochimica et Biophysica Acta. 2004. V. 1672. № 2. P. 100–111. https://doi.org/10.1016/j.bbagen.2004.03.002
Ромодин Л.А. Угнетение хлорофиллином хемилюминесценции, сопровождающей катализируемую комплексом цитохрома c с кардиолипином квазилипоксигеназную реакцию // Изв. Саратовского ун-та. Новая серия. Сер. Химия. Биология. Экология. 2020. Т. 20. № 4. С. 427–432. [Romodin LA. Chlorophyllin inhibits chemiluminescence that accompanies a quasi-hypoxygenase reaction catalyzed by the cytochrome c-cardiolipin complex // Izvestiya of Saratov University. Chemistry. Biology. Ecology. 2020. V. 20. № 4. P. 427–432. (In Russ.)]. https://doi.org/10.18500/1816-9775-2020-20-4-427-432
Кабакчи С.А., Архипов О.П., Лукашенко М.Л. Особенности радиолиза воды и водных растворов H2 и O2 при действии смешанного n,γ-излучения с высокой долей нейтронного компонента // Химия высоких энергий. 2013. Т. 47. № 4. С. 251–255. [Kabakchi S.A., Lukashenko M.L., Arkhipov O.P. Specific features of the radiolysis of water and aqueous solutions of H2 and O2 by mixed n,γ-radiation with a high portion of the neutron component // High Energy Chemistry. 2013. V. 47. № 4. P. 147–151]. https://doi.org/10.7868/S002311971304008X
Блохина Т.М., Иванов А.А., Воробьева Н.Ю. и др. Повреждение ДНК спленоцитов мышей при воздействии вторичного излучения, формирующегося при прохождении пучка 650 МэВ протонов через бетонную преграду // Бюл. эксперим. биологии и медицины. 2022. Т. 174. № 8. С. 154–159. [Blokhina T.M., Ivanov A.A., Vorobyeva N.Y., et al. DNA damage in splenocytes of mice exposed to se-condary radiation created by 650 MeV protons bombarding a concrete shielding barrier // Bull. Experim. Biology and Medicine. 2022. V. 174. № 2. P. 194–198]. https://doi.org/10.47056/0365-9615-2022-174-8-154-159
Wei J., Wang B., Wang H., et al. Radiation-Induced Normal Tissue Damage: Oxidative Stress and Epigenetic Mechanisms // Oxidative Medicine and Cellular Longevity. 2019. V. 2019. P. 3010342. https://doi.org/10.1155/2019/3010342
Henriquez-Hernandez L.A., Bordon E., Pinar B., et al. Prediction of normal tissue toxicity as part of the individualized treatment with radiotherapy in oncology patients // Surgical oncol. 2012. V. 21. № 3. P. 201–206. https://doi.org/10.1016/j.suronc.2011.12.002
Chadwick K.H., Leenhouts H.P. Radiation risk is linear with dose at low doses // Brit. J. Radiol. 2005. V. 78. № 925. P. 8–10. https://doi.org/10.1259/bjr/51173413
Osipov A.N., Smetanina N.M., Pustovalova M.V., et al. The formation of DNA single-strand breaks and alkali-labile sites in human blood lymphocytes exposed to 365-nm UVA radiation // Free Radical Biology & Medicine. 2014. V. 73. P. 34–40. https://doi.org/10.1016/j.freeradbiomed.2014.04.027
Korzeneva I.B., Kostuyk S.V., Ershova L.S., et al. Human circulating plasma DNA significantly decreases while lymphocyte DNA damage increases under chronic occupational exposure to low-dose gamma-neutron and tritium beta-radiation // Mutat. Res. 2015. V. 779. P. 1. P. 15. https://doi.org/10.1016/j.mrfmmm.2015.05.004
Olive P.L., Banath J.P., Durand R.E. Heterogeneity in radiation-induced DNA damage and repair in tumor and normal cells measured using the “comet” assay // Radiat. Res. 1990. V. 122. № 1. P. 86–94 // Methods In Molecular Biology. 2023. V. 2519. P. 65–72. https://doi.org/10.1007/978-1-0716-2433-3_7
Walsh K.D., Kato T.A. Alkaline Comet Assay to Detect DNA Damage // Methods in molecular biology. 2023. № 2519. Р. 65–72. https://doi.org/10.1007/978-1-0716-2433-3_7
Cooper C.R., Jones D.J.L., Jones G.D.D., Petersson K. Comet Assay Profiling of FLASH-Induced Damage: Mechanistic Insights into the Effects of FLASH Irradiation // Int. J. Molec. Sci. 2023. V. 24. № 8. P. 7195. https://doi.org/10.3390/ijms24087195
Dirven Y., Eide D.M., Henriksson E.W. et al. Assessing testicular germ cell DNA damage in the comet assay; introduction of a proof-of-concept // Environ. Molec. Mutagen. 2023. V. 64. № 2. P. 88–104. https://doi.org/10.1002/em.22527
Effat Saied N., Elmazny G.M., El-Helaly R.M. et al. Utility of comet assay of DNA damage in the detection of malignant transformation of chronic liver cirrhosis // Scand. J. Clin. Lab. Investig. 2023. V. 83. № 3. P. 145–151. https://doi.org/10.1080/00365513.2023.2175327
Tung G.K., Gandhi G. Baseline and oxidatively damaged DNA in end-stage renal disease patients on varied hemodialysis regimens: a comet assay assessment // Molec. Cell. Biochem. 2023;Online ahead of print. https://doi.org/10.1007/s11010-023-04720-4
Unverricht-Yeboah M., Holtmann K., Kriehuber R. Comet Assay analysis of DNA strand breaks after exposure to the DNA-incorporated Auger Electron Emitter Iodine-125 // Int. J. Radiat. Biol. 2023. V. 99. № 1. P. 64–69. https://doi.org/10.1080/09553002.2020.1851059
Goldoni A., Pacheco M.R., da Silva L.B. Comet assay in Aegla platensis (Decapoda: Anomura) using a non-lethal hemolymph field sampling for in situ monitoring of freshwater genotoxicity // Ecotoxicol. 2023. V. 32. № 2. P. 160–165. https://doi.org/10.1007/s10646-023-02627-w
Hasanovic M., Cetkovic T., Pourrut B., et al. Air pollution in Sarajevo, Bosnia and Herzegovina, assessed by plant comet assay // Mutagenesis. 2023. V. 38. № 1. P. 43–50. https://doi.org/10.1093/mutage/geac022
Karbaschi M., Ji Y., Mujawar M.A., Mendoza M. et al. Development of a Novel, Automated High-Throughput Device for Performing the Comet Assay // Int. J. Molec. Sci. 2023. V. 24. № 8. P. 7187. https://doi.org/10.3390/ijms24087187
Moller P., Azqueta A., Boutet-Robinet E., et al. Minimum Information for Reporting on the Comet Assay (MIRCA): recommendations for describing comet assay procedures and results // Nature Protocols. 2020. V. 15. № 12. P. 3817–3826. https://doi.org/10.1038/s41596-020-0398-1
Azqueta A., Ladeira C., Giovannelli L. et al. Application of the comet assay in human biomonitoring: An hCOMET perspective // Mutat. Res. Reviews in Mutation research. 2020. V. 783. P. 108288. https://doi.org/10.1016/j.mrrev.2019.108288
Сирота Н.П., Кузнецова Е.А. Применение метода “Комета тест” в радиобиологических исследованиях // Радиац. биология. Радиоэкология. 2010. Т. 50. № 3. С. 329–339. [Sirota N.P., Kuznecova E.A. The Comet Assay Application in Radiobiological Investigations // Radiacionnaya biologiya. Radioekologiya. 2010. V. 50. № 3. P. 329–339. (In Russ.)]
Мельниченко А.С. Математическая статистика и анализ данных: Учебное пособие. М.: Изд. дом НИТУ “МИСиС”, 2018, 45 с. [Mel’nichenko A.S. Matematicheskaya statistika i analiz dannyh: Uchebnoe posobie. M.: Izdatel’skij dom NITU “MISiS”, 2018, 45 p. (In Russ.)]
Abraham S.K., Sarma L., Kesavan P.C. Role of chlorophyllin as an in vivo anticlastogen: protection against gamma-radiation and chemical clastogens // Mutat. Res. 1994. V. 322. № 3. P. 209–212. https://doi.org/10.1016/0165-1218(94)90008-6
Zimmering S., Olvera O., Hernandez M.E. et al. Evidence for a radioprotective effect of chlorophyllin in Drosophila // Mutat. Res. 1990. V. 245. № 1. P. 47–49. https://doi.org/10.1016/0165-7992(90)90024-e
Lett J.T. Damage to cellular DNA from particulate radiations, the efficacy of its processing and the radiosensitivity of mammalian cells. Emphasis on DNA double strand breaks and chromatin breaks // Radiat. Environ. Biophys. 1992. V. 31. № 4. P. 257–277. https://doi.org/10.1007/BF01210207
Peak M.J., Peak J.G. Hydroxyl radical quenching agents protect against DNA breakage caused by both 365-nm UVA and by gamma radiation // Photochem. Photobiol. 1990. V. 51. № 6. P. 649–652.
Peak J.G., Ito T., Robb F.T., Peak M.J. DNA damage produced by exposure of supercoiled plasmid DNA to high- and low-LET ionizing radiation: effects of hydroxyl radical quenchers // Int. j. Radiat. Biol. 1995. V. 67. № 1. P. 1–6. https://doi.org/10.1080/09553009514550011
Векшин Н.Л. Биофизика митохондрий. Пущино: ООО “Фотон век”, 2019. 264 с. [Vekshin NL. Biofizika mitohondrij. Pushchino: OOO “Foton vek”; 2019. 264 p. (In Russ.)]
Дополнительные материалы отсутствуют.
Инструменты
Радиационная биология. Радиоэкология