Почвоведение, 2023, № 12, стр. 1628-1643
Содержание органического вещества и скорость разложения стандартного материала в почвах высокогорных фитоценозов Тебердинского национального парка
Т. Г. Елумеева a, *, М. И. Макаров a, М. С. Кадулин a, К. Н. Замалетдинова a, Т. И. Малышева a, Д. М. Гулов b, А. А. Ахметжанова a, М. А. Чепурнова a, В. Г. Онипченко a, c
a МГУ им. М.В. Ломоносова
119991 Москва, Ленинские горы, 1, Россия
b Башкирский государственный университет
450076 Уфа, ул. Валиди, 32, Россия
c Тебердинский национальный парк
369210 Теберда, Бадукский пер., 1, Россия
* E-mail: elumeeva@mail.ru
Поступила в редакцию 11.04.2023
После доработки 08.06.2023
Принята к публикации 21.06.2023
- EDN: ALUSMC
- DOI: 10.31857/S0032180X23600701
Аннотация
Высокогорные почвы очень разнообразны по содержанию органического вещества (ПОВ), но факторы, определяющие такое разнообразие, все еще полностью не выявлены. В 16 субальпийских, альпийских и субнивальных фитоценозах Тебердинского национального парка (северо-западный Кавказ) исследовали физико-химические и микробиологические характеристики почв, а также оценили параметры разложения стандартного материала на основе “индекса чайных пакетиков” (tea bag index, TBI): показатель стабилизации (STBI) и константу скорости разложения (kTBI). Проверяли следующие гипотезы: (1) ПОВ – один из предикторов STBI и kTBI в высокогорьях наряду с другими физико-химическими свойствами почвы; (2) больше ПОВ содержится при высоких значениях STBI и низких kTBI; (3) содержание ПОВ скоррелировано с подземной продукцией растений. Ведущими градиентами в изученных почвах являются: содержание влаги (автоморфные–гидроморфные почвы) и сопутствующее накопление ПОВ, а также высотный градиент, вверх по которому снижалось базальное дыхание почвы. Степень насыщения азотом (т.е. качество ПОВ) экстрагируемой фракции оказалась лучшим предиктором скорости разложения. Показатель STBI снижался при увеличении содержания общего углерода и потерь при прокаливании, а положительная связь kTBI с ПОВ отмечена только на автоморфных почвах. Таким образом, для богатых органическим веществом почв характерны низкий показатель стабилизации и относительно высокая скорость разложения. В фитоценозах с травянистыми доминантами содержание ПОВ было положительно скоррелировано с продукцией тонких корней, что подчеркивает важную роль продуктивности в накоплении органического вещества.
ВВЕДЕНИЕ
Накопление почвенного органического вещества (ПОВ) зависит от продуктивности растительного сообщества, которая определяется климатом и доступностью воды и элементов минерального питания, а также от баланса между стабилизацией (трансформацией соединений в более устойчивые) и разложением (минерализацией) [4, 12, 49, 56]. Высокая продуктивность может сочетаться с низким содержанием ПОВ, когда разложение идет быстрее, чем стабилизация (тропические леса), а в низкопродуктивных фитоценозах стабилизация может преобладать над разложением, вызывая накопление ПОВ (верховые болота) [28]. В альпийских тундрах почвы под относительно продуктивными кустарничковыми сообществами содержат меньше углерода, чем под травяными, что может объясняться более быстрым разложением органического вещества вследствие высокой активности микоризных грибов и формирования более благоприятного микроклимата для активности сапротрофов [55]. Кроме того, физические и химические свойства почвы контролируют количество содержащегося в них органического вещества, влияя на его доступность для микробного разложения [32, 53, 54].
Низкая скорость разложения обычно определяется факторами, отрицательно влияющими на ферментативные реакции: низкие температуры, дефицит влаги или анаэробные условия вследствие ее избытка [29, 46]. Если эти факторы удовлетворительны, возможны три основных механизма стабилизации ПОВ [46, 49]: (1) устойчивость поступающего органического вещества к разложению и его постепенное преобразование микроорганизмами в еще более устойчивое ПОВ, (2) физическая изоляция ПОВ от микроорганизмов в почвенных агрегатах, включая микроанаэробные зоны, (3) образование органо-минеральных соединений ПОВ с Al, Fe и Ca.
Индекс “чайных пакетиков” (tea bag index, TBI) позволяет стандартным методом сравнить скорость начальных стадий трансформации органического вещества в разных условиях [28]. При этом учитываются оба процесса: стабилизация и разложение. Показатель стабилизации (stabilization factor, STBI) оценивает, какая часть лабильной фракции органического вещества переходит в трудноразлагаемые соединения, а константа разложения (decomposition constant, kTBI) – насколько быстро идет минерализация.
Возможны разные комбинации этих показателей. Низкие значения STBI и высокие kTBI должны приводить к слабой аккумуляции органического вещества в почве. Наоборот, высокие значения STBI и низкие kTBI должны приводить к высокой аккумуляции. Хотя накопление ПОВ – процесс длительный, его количество в почве динамично и связано с актуальными условиями, которые могут поддерживать стабильность или, напротив, вызывать дестабилизацию органических соединений [4]. Таким образом, начальная скорость разложения стандартного материала может быть, по крайней мере, частично, связана с содержанием ПОВ. Однако, если значения и STBI, и kTBI оба низкие или оба высокие, накопление ПОВ может сильнее зависеть от продуктивности, когда почвы более продуктивных экосистем содержат больше органического вещества, а также от функциональных признаков доминирующих видов растений, определяющих устойчивость растительных остатков к разложению [22]. Таким образом, начальная скорость разложения не будет скоррелирована с ПОВ. В накоплении ПОВ подземная продукция может играть более важную роль, чем надземная [6]. На разложение корни влияют опосредовано, например, их выделения могут вызывать прайминг-эффект, при котором стимулируется разложение не только вновь поступившего органического вещества, но и ПОВ [6, 20], и в лесах удаление живых корней вызывает увеличение ПОВ в органо-минеральных комплексах [44]. Таким образом, при большом объеме тонких корней в почве ожидается меньшее содержание ПОВ. С другой стороны, корневые выделения, трансформированные микроорганизмами, могут разлагаться труднее [13], что приводит к накоплению ПОВ. В дополнение, качество поступающего органического вещества (видовая принадлежность, органы растений) и его устойчивость к разложению влияют на формирование сообщества микроорганизмов и их активность, обеспечивая так называемый “home advantage effect” для конкретного местообитания [57]. Использование стандартного материала для оценки трансформации органического вещества позволяет избежать этого эффекта и получить сравнимые данные между сообществами.
Высокогорья – удобный объект для экологических исследований, так как влияние климата на высотном градиенте дополняется выраженным влиянием мезорельефа. В результате разнообразные местообитания могут располагаться на компактной территории в пределах одного высотного пояса. Кроме того, высокогорные сообщества очень разнообразны по продуктивности и содержанию ПОВ [3, 9, 14, 16, 30, 37], которое трудно прогнозируется свойствами почвы и факторами почвообразования в горах [25]. В настоящей работе исследовали связи между содержанием ПОВ, STBI и kTBI в почвах разных фитоценозов в субальпийском, альпийском и субнивальном поясах Северо-Западного Кавказа. Проверяли следующие гипотезы: (1) ПОВ – один из предикторов STBI и kTBI в высокогорьях наряду с другими физико-химическими свойствами почвы; (2) больше ПОВ содержится при высоких значениях STBI и низких kTBI; (3) содержание ПОВ скоррелировано с подземной продукцией растений, которая влияет на его связи с параметрами разложения, оцененными с использованием TBI.
ОБЕКТЫ И МЕТОДЫ
Объекты исследования. Изученные участки располагались в субальпийском, альпийском и субнивальном поясах на горе Малая Хатипара в Тебердинском национальном парке (северо-западный Кавказ, Россия). Для района характерен типичный горный климат умеренной зоны. Среднегодовая температура в альпийском поясе составляет около –1.2°C, а среднегодовое количество осадков – 1400 мм/год [40]. В почвенном покрове преобладают почвы с органогенным или слаборазвитым гумусовым горизонтом, принадлежащие к отделу литоземов и петроземов по классификации почв России (2004) [2]. В аккумулятивных элементах рельефа встречаются почвы с торфяным горизонтом (торфяно-литоземы). Почвы сформированы на силикатных горных породах (серые граниты, слюдяные сланцы), в них отсутствуют карбонаты [1]. Почвы субнивальных и альпийских осыпей отличаются высокой каменистостью, достигающей 95 мас. %. В целом, если исключить крупные валуны, то уровень каменистости остальных автоморфных почв под альпийскими и субальпийскими растительными сообществами значительно ниже и находится в диапазоне 2–20%. Гранулометрический состав мелкозема почв субнивальных и альпийских осыпей песчаный и супесчаный, остальных автоморфных почв – легкосуглинистый. Верхняя граница леса находится на высоте около 2500 м над ур. м.
Изучали свойства почв 16 фитоценозов, типичных для трех поясов в диапазоне высот от 2180 до 3070 м над ур. м. В субальпийском поясе исследовали литоземы темногумусовые и перегнойно-темногумусовые [2] следующих фитоценозов: березняк рододендроновый (БР), сосняк вейниковый (СВ), субальпийское высокотравное сообщество (СубВ), субальпийский вейниковый луг (СВЛ), а также торфяно-литозем субальпийского болота (СубБ). В альпийском поясе литоземы были приурочены к таким фитоценозам как можжевельниковое сообщество (МС), пестрооовсяницевый луг (ПЛ), гераниево-копеечниковый луг (ГКЛ), альпийская лишайниковая пустошь (АЛП) и альпийский ковер (АК), а также литозем грубогумусовый в рододендроннике (РОД). Также в этом поясе исследовали торфяно-литозем альпийского болота (АБ) и петроземы гумусовые альпийской сухой осыпи (АСО) и альпийской влажной осыпи (АВО). В субнивальном поясе исследовали петроземы гумусовые субнивальной сухой осыпи (ССО) и субнивальной влажной осыпи (СВО).
Помимо разного высотного положения, почвы этих фитоценозов занимают разное положение в рельефе (от наветренных склонов до плоских оснований склонов) и дифференцируются по глубине и длительности снежного покрова. Подробное описание изученных сообществ приведено в монографии [39].
Эксперимент с разложением. В качестве стандартного материала для эксперимента с разложением использовали чай Lipton в пирамидках: зеленый (Camellia sinensis; EAN: 87 22700 05552 5) и ройбос (Aspalathus linearis; EAN: 87 22700 18843 8), в соответствии с модифицированным протоколом TBI [28] период инкубации был увеличен в связи с очень низкими значениями kTBI [17, 28, 58]. Наблюдения проводили в два сезона: с 18–19 сентября 2011 г. по 20–21 сентября 2012 г. (12 сообществ) и с 16–18 сентября 2015 г. по 13–14 сентября 2016 г. (8 сообществ). В четырех фитоценозах (АЛП, ГКЛ, АБ и СубБ) образцы были заложены в оба сезона.
В почву каждого фитоценоза на глубину 8 см закладывали пакетики зеленого чая и ройбоса в пятикратной повторности. Расстояние между пакетиками в паре и между повторностями составило 25 см. По окончании эксперимента рассчитывали потерю массы как разницу между исходной и итоговой массой зеленого чая или ройбоса в каждом пакетике. Перед взвешиванием содержимого из пакетиков удаляли вросшие корни и высушивали образцы при 80°C в течение 24 ч. После взвешивания образцы прокаливали при 500°C, и массу золы вычитали из сухой массы, чтобы исключить загрязнение образцов почвой (доля золы, исходно содержащейся в чае, очень мала и составляет 0.2–0.4% [28]).
Факторы среды, влияющие на разложение. Для характеристики условий разложения использовали абсолютную высоту, которая отражает изменение температурного режима на исследованных участках. Почвы считались промерзающими, если снежный покров держится только часть зимы, а его глубина составляет менее 0.5 м [39]. Характеристики снежного покрова не связаны с высотой на изученном градиенте, так как накопление снега в горах в большей степени зависит от рельефа и направления ветра [40].
Методы измерения свойств почв. Для сравнительной характеристики обеспеченности почв влагой определяли массовую влажность. Пробы отбирали в течение одних суток в августе спустя три дня после дождя. К этому времени снежный покров полностью сошел на всех участках, и в почву не поступало талой воды. Хотя влажность почвы сильно зависит от сезонной и разногодичной изменчивости количества осадков и интенсивности эвапотранспирации, однократное измерение может характеризовать градиент влажности в почвах изученных сообществ.
Для оценки глубины залегания скального основания использовали стальной щуп длиной 35 см.
Отбор образцов почвы проводили в пятикратной повторности из слоя 0–10 см верхнего горизонта (W, AU, AO, AH, T) вблизи точек заложения стандартного материала. В автоморфных почвах верхний органогенный горизонт предварительно удаляли. Образцы почвы замораживали в течение 5 ч с момента отбора и хранили в таком состоянии до начала лабораторных исследований.
Определение минералогического состава автоморфных почв проводили иммерсионным методом из одной смешанной пробы. Для каждой пробы рассматривали по 500 зерен фракции 0.25–1 мм. Выделяли три группы минералов: кварц, полевые шпаты, а также основные минералы (слюды, роговая обманка, пироксены и др.).
Для характеристики микробиологической активности почвы, а также содержания лабильных форм углерода и азота использовали образцы почв естественной влажности (без высушивания) сразу после разморозки.
При подготовке влажные образцы минеральных горизонтов почв пропускали через сито с диаметром отверстий 5 мм и вручную выбирали корни и камни. Петроземы сразу просеивали через сито с диаметром отверстий 2 мм. Влажные образцы торфа измельчали ножницами.
Из влажных образцов получали солевые вытяжки (0.05 M K2SO4) при соотношении почва : раствор 1 : 5 и фильтрации через бумажный фильтр “синяя лента”.
Содержание экстрагируемого органического углерода (Сорг-экстр) и общего экстрагируемого азота (Nэкстр) в вытяжках измеряли при помощи жидкостного анализатора Shimadzu TOC(N)-VCPN.
Неорганические формы азота определяли колориметрически: аммонийный азот (N–NH4) – салицилат-нитропрусидным методом [27]; нитратный азот (N–NO3) – при восстановлении до NO2 на кадмиевой колонке и его последующим окрашиванием сульфаниламидом и N-(1-нафтил)-этилендиамин-дигидрохлоридом [15].
Органический экстрагируемый азот (Nорг-экстр) рассчитывали как разницу между его общим содержанием в вытяжке (Nэкстр) и суммой минеральных форм (N–NH4 + N–NO3).
Интенсивность N-минерализации (накопление минеральных форм азота при разложении органического вещества почвы) оценивали посредством инкубации почвы при условно полевой влажности в течение 21 сут при 22°С. Постоянную температуру поддерживали в термостате Sanyo MIR-154. Контроль влажности почвы осуществляли весовым методом.
Базальное дыхание почвы с условно полевой влажностью измеряли на газовом хроматографе Кристалл 2000 при накоплении CO2 в течение 1 сут при 22°С.
Остальные показатели определяли при стандартной процедуре подготовки проб сухой почвы. ${\text{p}}{{{\text{H}}}_{{{{{\text{H}}}_{{\text{2}}}}{\text{O}}}}}$ измеряли в водной вытяжке 1 : 5. Неорганический фосфор (Pподв) из почвы экстрагировали по Кирсанову с последующим окрашиванием по Мэрфи и Райли. Колориметрирование аммонийного и нитратного азота, а также фосфора осуществляли на спектрофотометре Genesys 10uv.
Для оценки содержания ОВ в почвах наряду с классическим методом потери при прокаливании (ППП) применяли метод сухого сжигания с помощью элементного CN анализатора Elementar Vario EL III. Использовали образцы почв, размолотые до пудры на шаровой мельнице Retsch MM200. Для определения ППП образцы почвы массой от 3 до 10 г брали в каждом сообществе в трехкратной повторности. Образцы высушивали в сушильном шкафу при 105°C в течение 12 ч, а затем прокаливали в муфельной печи при 500°C в течение 8 ч. Эти два метода позволяют получать сопоставимые результаты определения содержания органического вещества в бескарбонатных сходных по генезису горизонтах (гумусовые и органогенные, как в настоящем случае) [23].
Продукцию тонких корней использовали в качестве показателя подземной продуктивности растений. Ее определяли с использованием чайных ситечек из нержавеющей стали с размером ячейки около 1.5 мм и диаметром 4.5 см (объем 47.7 см3), заполненных просеянной почвой и закопанных на глубину около 7 см в пятикратной повторности в каждом сообществе [41]. Время экспозиции составило около 60 дней и охватывало вторую часть вегетационного периода в лесном поясе, с 17–20 июля по 19–20 сентября 2019 г. После выкапывания вросшие корни отделяли от почвы, промывали, высушивали в сушильном шкафу при 80°C в течение 10 ч и взвешивали. Эти измерения дают сравнительную оценку продукции корней между сообществами за один и тот же промежуток времени.
Анализ данных. Скорость разложения kTBI и показатель стабилизации STBI оценивали, исходя из потерь массы зеленым чаем и ройбосом по методике [28]. STBI был рассчитывали по формуле S = = 1 – ag/Hg, где ag – отношение между потерей массы и исходной массой образца зеленого чая, а Hg – стандартная величина его лабильной фракции, равная 0.842 г/г. Для вычисления kTBI применяли экспоненциальные модели по формуле ar(t) = are–kt, где ar(t) – масса лабильной фракции ройбоса, не разложившейся за период наблюдений, а ar – общая величина лабильной фракции с учетом показателя стабилизации. Для каждого образца ройбоса ar(t) рассчитывали как ar(t) = = Wr(t) – (1 – ar), где Wr(t) – масса образца ройбоса после окончания опыта. Общую величину лабильной фракции ройбоса (ar) оценивали по формуле ar = Hr (1 – S), где Hr – стандартная величина лабильной фракции ройбоса, равная 0.552 г/г [28]. При вычислении kTBI допускали, что только лабильная фракция разлагается в течение года инкубации.
Чтобы сравнить значения STBI и kTBI, полученные в разные годы в четырех сообществах (АЛП, ГКЛ, АБ и СубБ), использовали непараметрический тест Манна–Уитни.
Для всех признаков почв, а также для высоты над уровнем моря, рассчитывали коэффициенты корреляции Спирмена. Провели ординацию сообществ по свойствам почв методом главных компонент в пакете vegan [38]. Использовали следующие показатели: массовая влажность, pH, Cобщ, Nобщ, N–NH4, N–NO3, Pподв, базовое дыхание почвы и скорость минерализации азота. Данные для ординации были стандартизованы. Коэффициенты корреляции STBI и kTBI с двумя первыми осями наибольшего варьирования рассчитывали с помощью перестановочных тестов, функция “envfit” в пакете vegan. Так как в ходе ординации гидроморфные почвы болот резко выделялись среди остальных, то дополнительно провели ординацию для 14 сообществ, исключив болота. Для них дополнительно в анализ включили процентное содержание минералов (полевые шпаты, кварц и основные минералы).
Для выявления связи STBI и kTBI с факторами среды использовали регрессионные модели со смешанным эффектом с помощью функции “lme” в пакете nlme [43] в статистической среде R 4.1.0 [50]. В качестве фиксированных эффектов в модели были включены высота и все измеренные физико-химические и микробиологические свойства, а сообщество рассматривали как случайный эффект для того, чтобы учесть варьирование между образцами в пределах одной повторности. Лучшую модель отбирали в ходе автоматической пошаговой регрессии (функция “stepAIC”). Итоговые модели проверяли на нормальное распределение ошибок по квантиль-квантильным диаграммам, а также на фактор инфляции дисперсии, функция “vif” в пакете car в R [21]. Значимость фиксированных эффектов оценивали с помощью анализа отклонений II типа с помощью функции “Anova” в пакете car. Коэффициенты детерминации дисперсии, объясненной фиксированными эффектами, (marginal R2) рассчитывали в пакете MuMIn [5, 36].
Чтобы выяснить, может ли содержание ПОВ быть предсказано показателем стабилизации и скоростью разложения и влияет ли продуктивность корней на эти взаимоотношения, мы провели такой же анализ с ППП и Собщ в качестве зависимых переменных и STBI, kTBI и продуктивностью корней как предикторами. В этом случае для STBI и kTBI были взяты средние значения за все годы. Дополнительно построили модель только для сообществ с травянистыми доминантами, так как корневая продуктивность древесных сообществ обычно меньше по сравнению с травяными фитоценозами, поскольку древесные растения увеличивают поверхность поглощения за счет гиф микоризных симбионтов, что не отражает корневую продуктивность [10, 34].
РЕЗУЛЬТАТЫ И ОБСУЖДЕНИЕ
Разнообразие горных почв Тебердинского национального парка. Почвы под высокогорными фитоценозами Тебердинского национального парка разнообразны по физико-химическим свойствам и микробиологической активности (табл. 1–2). Глубина залегания скального основания в автоморфных почвах варьировала от 8.3–8.4 см на осыпях до 15–20 см на пологих склонах (МС, ПЛ), а в гидроморфных почвах за счет накопления органического материала порода была глубже 35 см.
Таблица 1.
Общая характеристика физико-химических свойств почвы. Жирным шрифтом выделены сообщества с древесными доминантами. Приведены средние (над чертой) и ошибки среднего (под чертой). n = 5 для всех параметров, кроме потерь при прокаливании (ППП). БР, березняк рододендоновый; СВ, сосняк вейниковый; СубБ, субальпийское болото; СубВ, субальпийское высокотравное сообщество; СВЛ, субальпийский вейниковый луг; МС, можжевеловый стланик; ПЛ, пестроовсяницевый луг; РОД, рододендронник; ГКЛ, гераниево-копеечниковый луг; АЛП, альпийская лишайниковая пустошь; АК, альпийский ковер; АВО, альпийская влажная осыпь; АБ, альпийское болото; АСО, альпийская сухая осыпь; ССО, субнивальная сухая осыпь; СВО, субнивальная влажная осыпь
Сообщество | Высота, м над ур. м. | Почва | Горизонт | Промерзание почвы (+/–) | Глубина залегания камня, см | Массовая влажность, % | ${\text{p}}{{{\text{H}}}_{{{{{\text{H}}}_{{\text{2}}}}{\text{O}}}}}$ | P, мг/кг | Cобщ, % | Nобщ, % | C : Nобщ | ППП, % (n = 3) |
---|---|---|---|---|---|---|---|---|---|---|---|---|
БР | 2184 | Литозем перегнойно-темногумусовый | AH | – | $\frac{{16.4}}{{1.0}}$ | $\frac{{44.6}}{{6.6}}$ | $\frac{{4.13}}{{0.11}}$ | $\frac{{169.2}}{{34.8}}$ | $\frac{{16.1}}{{2.45}}$ | $\frac{{0.8}}{{0.11}}$ | $\frac{{19.8}}{{0.84}}$ | $\frac{{68.4}}{{5.9}}$ |
СВ | 2422 | Литозем темногумусовый | AU | + | $\frac{{13.9}}{{0.9}}$ | $\frac{{21.8}}{{9.6}}$ | $\frac{{5.14}}{{0.08}}$ | $\frac{{111.1}}{{7.0}}$ | $\frac{{11.2}}{{2.82}}$ | $\frac{{0.6}}{{0.09}}$ | ![]() |
$\frac{{11.3}}{{1.1}}$ |
СубБ | 2541 | Торфяно-литозем | T1 | – | >35 | $\frac{{606.7}}{{19.7}}$ | $\frac{{5.93}}{{0.17}}$ | $\frac{{213.5}}{{25.5}}$ | $\frac{{40.5}}{{0.77}}$ | $\frac{{1.7}}{{0.11}}$ | $\frac{{23.9}}{{1.93}}$ | $\frac{{90.1}}{{0.7}}$ |
СубВ | 2542 | Литозем темногумусовый | AU | – | $\frac{{13.8}}{{1.3}}$ | $\frac{{32.7}}{{2.4}}$ | $\frac{{4.90}}{{0.12}}$ | $\frac{{30.4}}{{1.6}}$ | $\frac{{9.9}}{{0.48}}$ | $\frac{{0.9}}{{0.05}}$ | $\frac{{10.5}}{{0.21}}$ | $\frac{{27.5}}{{2.8}}$ |
СВЛ | 2550 | Литозем темногумусовый | AU | – | $\frac{{16.0}}{{0.8}}$ | $\frac{{49.1}}{{11.3}}$ | $\frac{{4.93}}{{0.07}}$ | $\frac{{19.3}}{{3.2}}$ | $\frac{{13.7}}{{2.01}}$ | $\frac{{1.0}}{{0.12}}$ | $\frac{{13.2}}{{0.43}}$ | $\frac{{26.8}}{{2.2}}$ |
МС | 2701 | Литозем перегнойно-темногумусовый | AH | + | $\frac{{18.6}}{{1.3}}$ | $\frac{{106.5}}{{9.5}}$ | $\frac{{5.09}}{{0.08}}$ | $\frac{{13.3}}{{1.6}}$ | $\frac{{18.0}}{{1.47}}$ | $\frac{{1.2}}{{0.11}}$ | $\frac{{15.0}}{{0.25}}$ | $\frac{{30.2}}{{3.9}}$ |
ПЛ | 2706 | Литозем темногумусовый | AU | – | $\frac{{19.6}}{{0.8}}$ | $\frac{{60.9}}{{9.9}}$ | $\frac{{5.15}}{{0.03}}$ | $\frac{{12.2}}{{1.2}}$ | $\frac{{13.2}}{{1.2}}$ | $\frac{{1.0}}{{0.81}}$ | $\frac{{13.5}}{{0.07}}$ | $\frac{{28.7}}{{2.1}}$ |
РОД | 2750 | Литозем грубогумусовый | AO | – | $\frac{{14.5}}{{1.3}}$ | $\frac{{146.7}}{{20.5}}$ | $\frac{{4.42}}{{0.14}}$ | $\frac{{22.7}}{{6.0}}$ | $\frac{{31.3}}{{3.75}}$ | $\frac{{1.3}}{{0.10}}$ | $\frac{{24.2}}{{1.03}}$ | $\frac{{77.6}}{{12.8}}$ |
ГКЛ | 2751 | Литозем темногумусовый | AU | – | $\frac{{15.0}}{{0.9}}$ | $\frac{{40.1}}{{3.6}}$ | $\frac{{4.75}}{{0.04}}$ | $\frac{{13.5}}{{0.5}}$ | $\frac{{8.7}}{{0.97}}$ | $\frac{{0.7}}{{0.05}}$ | $\frac{{12.4}}{{0.55}}$ | $\frac{{20.7}}{{3.1}}$ |
АЛП | 2780 | Литозем темногумусовый | AU | + | $\frac{{16.7}}{{1.2}}$ | $\frac{{86.5}}{{9.4}}$ | $\frac{{5.64}}{{0.03}}$ | $\frac{{2.6}}{{0.3}}$ | $\frac{{10.8}}{{1.45}}$ | $\frac{{0.8}}{{0.10}}$ | $\frac{{12.8}}{{0.30}}$ | $\frac{{23.3}}{{0.7}}$ |
АК | 2789 | Литозем перегнойно-темногумусовый | AH | – | $\frac{{17.2}}{{1.5}}$ | $\frac{{95.8}}{{4.5}}$ | $\frac{{4.89}}{{0.02}}$ | $\frac{{9.5}}{{1.4}}$ | $\frac{{15.0}}{{1.95}}$ | $\frac{{1.2}}{{0.15}}$ | $\frac{{12.9}}{{0.29}}$ | $\frac{{21.1}}{{0.9}}$ |
АВО | 2795 | Петрозем | M | – | $\frac{{8.3}}{{1.0}}$ | $\frac{{20.8}}{{4.5}}$ | $\frac{{5.12}}{{0.01}}$ | $\frac{{42.5}}{{2.3}}$ | $\frac{{2.3}}{{0.22}}$ | $\frac{{0.2}}{{0.02}}$ | $\frac{{9.4}}{{0.44}}$ | $\frac{{7.3}}{{0.6}}$ |
АБ | 2800 | Торфяно-литозем | T1 | – | >35 | $\frac{{627.4}}{{54.3}}$ | $\frac{{5.47}}{{0.07}}$ | $\frac{{143.5}}{{16.6}}$ | $\frac{{39.0}}{{0.84}}$ | $\frac{{2.1}}{{0.10}}$ | $\frac{{18.7}}{{1.22}}$ | $\frac{{75.4}}{{6.5}}$ |
АСО | 2899 | Петрозем | M | + | $\frac{{8.4}}{{0.6}}$ | $\frac{{4.2}}{{0.6}}$ | $\frac{{6.15}}{{0.10}}$ | $\frac{{51.5}}{{1.7}}$ | $\frac{{0.4}}{{0.06}}$ | $\frac{{0.1}}{{0.01}}$ | $\frac{{5.8}}{{0.46}}$ | $\frac{{5.2}}{{0.9}}$ |
ССО | 3063 | Петрозем | M | + | $\frac{{11.7}}{{1.1}}$ | $\frac{{4.0}}{{0.4}}$ | $\frac{{6.84}}{{0.05}}$ | $\frac{{128.4}}{{4.7}}$ | $\frac{{0.3}}{{0.01}}$ | $\frac{{0.1}}{{0.00}}$ | $\frac{{5.7}}{{0.48}}$ | $\frac{{4.3}}{{0.3}}$ |
СВО | 3069 | Петрозем | M | – | $\frac{{19.9}}{{1.4}}$ | $\frac{{9.3}}{{1.3}}$ | $\frac{{5.16}}{{0.02}}$ | $\frac{{50.2}}{{1.7}}$ | $\frac{{0.5}}{{0.03}}$ | $\frac{{0.1}}{{0.00}}$ | $\frac{{5.9}}{{0.14}}$ | $\frac{{4.3}}{{0.3}}$ |
Таблица 2.
Содержание экстрагируемых форм углерода и азота в почве и микробиологические параметры. Жирным шрифтом выделены сообщества с древесными растениями. Приведены средние ± ошибки среднего, n = 5 для всех параметров. Названия сообществ приведены в табл. 1
Сообщество | Сорг-экстр, мг/кг | Nорг-экстр, мг/кг | C : Nорг-экстр, мг/кг | N–NH4, мг/кг | N–NO3, мг/кг | C : Nорг-экстр/ C : Nобщ | Смикр, мг/кг | БД, мг C–CO2/(кг ч) | N-минерализация, мг N/(кг сут) |
---|---|---|---|---|---|---|---|---|---|
БР | $\frac{{394}}{{38}}$ | $\frac{{55}}{6}$ | $\frac{{7.3}}{{0.4}}$ | $\frac{{29.1}}{{2.1}}$ | $\frac{{0.1}}{{0.0}}$ | $\frac{{0.4}}{{}}$ | $\frac{{412.9}}{{53.5}}$ | $\frac{{7.77}}{{0.59}}$ | $\frac{{5.98}}{{0.40}}$ |
СВ | $\frac{{265}}{{43}}$ | $\frac{{21}}{4}$ | $\frac{{13.4}}{{0.8}}$ | $\frac{{29.2}}{{6.5}}$ | $\frac{{0.3}}{{0.2}}$ | $\frac{{0.7}}{{}}$ | $\frac{{351.1}}{{36.8}}$ | $\frac{{4.21}}{{1.04}}$ | $\frac{{0.67}}{{0.08}}$ |
СубБ | $\frac{{2198}}{{232}}$ | $\frac{{43}}{5}$ | $\frac{{39.5}}{{3.8}}$ | $\frac{{158.2}}{{24.0}}$ | $\frac{{4.5}}{{0.4}}$ | $\frac{{1.7}}{{}}$ | $\frac{{4203.5}}{{35.0}}$ | $\frac{{5.89}}{{0.37}}$ | $\frac{{ - 5.51}}{{0.83}}$ |
СубВ | $\frac{{310}}{{56}}$ | $\frac{{36}}{8}$ | $\frac{{9.7}}{{1.2}}$ | $\frac{{49.7}}{{8.2}}$ | $\frac{{0.7}}{{0.2}}$ | $\frac{{0.9}}{{}}$ | $\frac{{549.6}}{{45.7}}$ | $\frac{{6.67}}{{0.79}}$ | $\frac{{5.33}}{{0.82}}$ |
СВЛ | $\frac{{745}}{{91}}$ | $\frac{{74}}{8}$ | $\frac{{10.0}}{{0.6}}$ | $\frac{{33.7}}{{7.7}}$ | $\frac{{0.1}}{{0.0}}$ | $\frac{{0.8}}{{}}$ | $\frac{{963.6}}{{201.2}}$ | $\frac{{6.21}}{{1.12}}$ | $\frac{{4.70}}{{0.66}}$ |
МС | $\frac{{279}}{{12}}$ | $\frac{{37}}{2}$ | $\frac{{7.6}}{{0.2}}$ | $\frac{{33.8}}{{1.1}}$ | $\frac{{0.2}}{{0.0}}$ | $\frac{{0.5}}{{}}$ | $\frac{{1128.2}}{{127.7}}$ | $\frac{{3.82}}{{0.37}}$ | $\frac{{1.82}}{{0.18}}$ |
ПЛ | $\frac{{345}}{{45}}$ | $\frac{{46}}{7}$ | $\frac{{7.5}}{{0.3}}$ | $\frac{{24.0}}{{3.0}}$ | $\frac{{0.1}}{{0.0}}$ | $\frac{{0.6}}{{}}$ | $\frac{{955.8}}{{173.9}}$ | $\frac{{3.12}}{{0.36}}$ | $\frac{{3.53}}{{0.31}}$ |
РОД | $\frac{{789}}{{160}}$ | $\frac{{65}}{{11}}$ | $\frac{{11.7}}{{0.6}}$ | $\frac{{18.6}}{{2.8}}$ | $\frac{{0.1}}{{0.0}}$ | $\frac{{0.5}}{{}}$ | $\frac{{579.2}}{{146.2}}$ | $\frac{{6.32}}{{1.06}}$ | $\frac{{ - 0.48}}{{0.18}}$ |
ГКЛ | $\frac{{348}}{{23}}$ | $\frac{{49}}{2}$ | $\frac{{7.0}}{{0.2}}$ | $\frac{{28.2}}{{0.9}}$ | $\frac{{1.4}}{{0.9}}$ | $\frac{{0.6}}{{}}$ | $\frac{{619.9}}{{24.5}}$ | $\frac{{3.15}}{{0.10}}$ | $\frac{{5.00}}{{0.44}}$ |
АЛП | $\frac{{119}}{{10}}$ | $\frac{{10}}{1}$ | $\frac{{11.8}}{{0.6}}$ | $\frac{{22.3}}{{2.5}}$ | $\frac{{1.3}}{{0.3}}$ | $\frac{{0.9}}{{}}$ | $\frac{{604.8}}{{66.5}}$ | $\frac{{1.82}}{{0.12}}$ | $\frac{{ - 0.46}}{{0.10}}$ |
АК | $\frac{{396}}{{37}}$ | $\frac{{42}}{4}$ | $\frac{{9.5}}{{0.4}}$ | $\frac{{24.7}}{{2.5}}$ | $\frac{{0.6}}{{0.0}}$ | $\frac{{0.7}}{{}}$ | $\frac{{771.1}}{{81.6}}$ | $\frac{{3.40}}{{0.45}}$ | $\frac{{3.89}}{{0.23}}$ |
АВО | $\frac{{88}}{8}$ | $\frac{6}{1}$ | $\frac{{14.8}}{{1.8}}$ | $\frac{{4.9}}{{0.7}}$ | $\frac{{0.7}}{{0.0}}$ | $\frac{{1.6}}{{}}$ | $\frac{{32.3}}{{5.8}}$ | $\frac{{0.28}}{{0.05}}$ | $\frac{{0.64}}{{0.19}}$ |
АБ | $\frac{{4174}}{{645}}$ | $\frac{{232}}{{51}}$ | $\frac{{20.4}}{{2.7}}$ | $\frac{{169.3}}{{31.6}}$ | $\frac{{11.5}}{{0.8}}$ | $\frac{{1.1}}{{}}$ | $\frac{{2731.0}}{{367.2}}$ | $\frac{{1.16}}{{0.18}}$ | $\frac{{ - 4.05}}{{0.64}}$ |
АСО | $\frac{{36}}{2}$ | $\frac{3}{0}$ | $\frac{{13.1}}{{2.2}}$ | $\frac{{2.2}}{{0.2}}$ | $\frac{{1.3}}{{0.1}}$ | $\frac{{2.2}}{{}}$ | $\frac{{30.9}}{{5.1}}$ | $\frac{{0.02}}{{0.00}}$ | $\frac{{0.09}}{{0.01}}$ |
ССО | $\frac{{25}}{1}$ | $\frac{2}{0}$ | $\frac{{12.8}}{{1.4}}$ | $\frac{{0.9}}{{0.1}}$ | $\frac{{2.2}}{{0.1}}$ | $\frac{{2.2}}{{}}$ | $\frac{{11.7}}{{1.4}}$ | $\frac{{0.03}}{{0.01}}$ | $\frac{{0.09}}{{0.01}}$ |
СВО | $\frac{{31}}{1}$ | $\frac{2}{0}$ | $\frac{{16.0}}{{1.5}}$ | $\frac{{1.4}}{{0.2}}$ | $\frac{{0.8}}{{0.1}}$ | $\frac{{2.7}}{{}}$ | $\frac{{8.1}}{{1.2}}$ | $\frac{{0.26}}{{0.18}}$ | $\frac{{0.08}}{{0.01}}$ |
В исследованных автоморфных почвах основная доля минералов приходилась на кварц (24–50%) и группу полевых шпатов (29–56%). Число зерен основных минералов также было весьма значительным (16–29%). Однако темноцветных минералов практически не обнаружено, встречались лишь их единичные зерна. Таким образом, почвообразующие породы всех исследованных автоморфных почв в целом можно отнести к группе гранитов и гранодиоритов.
Массовая влажность изменялась от 4% на сухих осыпях до более 600% в гидроморфных почвах. Она не была связана с высотным градиентом, но отражала дифференциацию почв в элементах рельефа, и большинство измеренных признаков, кроме ${\text{p}}{{{\text{H}}}_{{{{{\text{H}}}_{{\text{2}}}}{\text{O}}}}}$ и БД, были с ней скоррелированы (табл. 3). В автоморфных почвах массовая влажность была положительно скоррелирована с содержанием кварцев (R = 0.688, p = 0.008) и отрицательно – с содержанием полевых шпатов (R = –0.758, p = 0.002). Диапазон кислотности охватывал как слабокислые и нейтральные значения ${\text{p}}{{{\text{H}}}_{{{{{\text{H}}}_{{\text{2}}}}{\text{O}}}}}$ (5.12–6.84) начальной стадии выветривания скальной породы на субнивальных и альпийских осыпях, так и низкие значения ${\text{p}}{{{\text{H}}}_{{{{{\text{H}}}_{{\text{2}}}}{\text{O}}}}}$ (4.13–4.42) в присутствии Rhododendron caucasicum Pall.
Таблица 3.
Коэффициенты корреляции Спирмена между свойствами почв альпийских сообществ (n = 16). Жирным курсивом выделены значения коэффициентов на уровне значимости p < 0.05, жирным шрифтом – p < 0.01
Параметр | Массовая влажность | pH | P | Cобщ | Nобщ | C : Nобщ | ППП | Cорг-экстр | Nорг-экстр | C : Nорг-экстр | N–NH4 | N–NO3 | Смикр | БД | N-минер |
---|---|---|---|---|---|---|---|---|---|---|---|---|---|---|---|
Высота | –0.090 | 0.564 | –0.322 | –0.344 | –0.355 | –0.630 | –0.459 | –0.089 | –0.149 | 0.026 | –0.244 | 0.111 | –0.230 | –0.833 | –0.345 |
Влажность | 0.178 | 0.581 | 0.878 | 0.819 | 0.590 | 0.766 | 0.933 | 0.703 | 0.773 | 0.962 | 0.859 | 0.936 | 0.100 | –0.712 | |
pH | 0.283 | –0.173 | –0.237 | –0.422 | –0.275 | 0.080 | –0.186 | 0.431 | 0.116 | 0.259 | 0.151 | –0.630 | –0.569 | ||
P | 0.431 | 0.238 | 0.404 | 0.523 | 0.515 | 0.260 | 0.675 | 0.597 | 0.486 | 0.539 | 0.143 | –0.485 | |||
Cобщ | 0.937 | 0.865 | 0.926 | 0.820 | 0.704 | 0.548 | 0.840 | 0.619 | 0.854 | 0.466 | –0.490 | ||||
Nобщ | 0.750 | 0.813 | 0.806 | 0.774 | 0.373 | 0.832 | 0.621 | 0.823 | 0.467 | –0.303 | |||||
C : Nобщ | 0.862 | 0.523 | 0.464 | 0.352 | 0.573 | 0.276 | 0.611 | 0.676 | –0.268 | ||||||
ППП | 0.706 | 0.611 | 0.483 | 0.732 | 0.497 | 0.741 | 0.582 | –0.362 | |||||||
Cорг-экстр | 0.896 | 0.578 | 0.928 | 0.939 | 0.802 | 0.066 | –0.581 | ||||||||
Nорг-экстр | 0.163 | 0.730 | 0.819 | 0.546 | 0.140 | –0.240 | |||||||||
C : Nорг-экстр | 0.715 | 0.547 | 0.780 | –0.033 | –0.871 | ||||||||||
N–NH4 | 0.851 | 0.934 | 0.213 | –0.568 | |||||||||||
N–NO3 | 0.675 | –0.220 | –0.631 | ||||||||||||
Смикр | 0.268 | –0.581 | |||||||||||||
БД | 0.372 |
Наибольшие значения ППП были характерны для почв болот (СубБ – 90.1%; АБ – 75.4%) и двух сообществ с высоким участием рододендрона (РОД – 77.6%; БР – 68.4%), а наименьшие – в почвах субнивальных осыпей (4.3%). Соответственно, на петроземах субнивальных и альпийских осыпей было наименьшее содержание Собщ (0.3–2.3%) и Nобщ (0.1–0.2%), а наибольшее содержание Собщ было закономерно приурочено к торфяным горизонтам T болотных почв (39.5–40.5%) так же, как относительно высокое содержание азота по сравнению с другими сообществами (1.7–2.1%). Содержание подвижного фосфора варьировало от 2.6 мг/кг в почвах альпийских пустошей до 213 мг/кг на субальпийских болотах. При этом в почвах субнивальных и альпийских осыпей концентрация Pподв составила 42.5–128.4 мг/кг, что можно объяснить его накоплением при выветривании скальной породы на фоне неблагоприятных условий для геохимической миграции (слабокислый и нейтральный ${\text{p}}{{{\text{H}}}_{{{{{\text{H}}}_{{\text{2}}}}{\text{O}}}}}$), а также крайне малым количеством корней растений, которые бы могли изымать этот элемент из почвы.
Содержание экстрагируемых форм органического углерода и азота в почвах с развитием растительного покрова возрастает при переходе от субнивальных и альпийских осыпей (25–88 и 2–6 мг/кг) к АЛП (119 и 10 мг/кг соответственно). Далее в целом для лесных и луговых сообществ альпийского и субальпийского пояса содержание Сорг-экстр (265–394 мг/кг) и Nорг-экстр (21–55 мг/кг) варьирует в довольно узком диапазоне, достигая максимума в почве РОД и СВЛ 745–789 и 65–74 мг/кг соответственно. Наибольшее содержание Сорг-экстр (2198–4174 мг/кг) было закономерно приурочено к торфяному горизонту болотных почв. При накоплении ОВ в почвах в ряду: осыпи – лесные и луговые сообщества – болота также возрастает содержание Cмикр (от 8–32 до 351–1128 и 2731–4203 мг/кг соответственно), N–NO3 (от 0.7–1.3 до 0.1–1.4 и 4.5–11.5 мг/кг) и N–NH4 (от 0.9–4.9 до 18.6–33.8 и 158.2–169.3 мг/кг).
Выделенные две первые главные компоненты объясняли 82.3% варьирования свойств почвы (рис. 1a). Первая ось объясняла 56.4% дисперсии и была связана с градиентом влажности почвы, содержанием углерода и азота. На вторую ось приходилось 25.9% дисперсии, вдоль этой оси снижается БД и увеличивается pH, таким образом, она отражает высотный градиент. STBI и kTBI были значимо скоррелированы с обеими осями, и связь с STBI определялась положением болот. При исключении болот из анализа и дополнении данными о минералогическом составе, уже первая ось (50.2% варьирования) показывала высотный градиент, по которому в автоморфных почвах увеличивались pH и N–NO3 и уменьшались БД, Cобщ, Nобщ, N–NH4 (рис. 1b). С этой осью была скоррелирована kTBI, а STBI значимо не коррелировал ни с одной из осей, хотя и имел тенденцию снижаться вдоль второй оси. По второй оси (20.4% варьирования) возрастало содержание Pподв и уменьшалась доля основных минералов.
Рис. 1.
Ординационные диаграммы свойств почв высокогорных сообществ методом главных компонент (PCA): a – все сообщества, b – без альпийского и субальпийского болота. PC1, PC2 – две первые оси наибольшего варьирования. Сокращения названий сообществ см. в табл. 1. Почвы: 1 – литоземы темногумусовые; 2 – литоземы перегнойно-темногумусовые и литозем грубогумусовый; 3 – петроземы гумусовые; 4 – торфяно-литоземы. const – константа скорости разложения. S – показатель стабилизации.
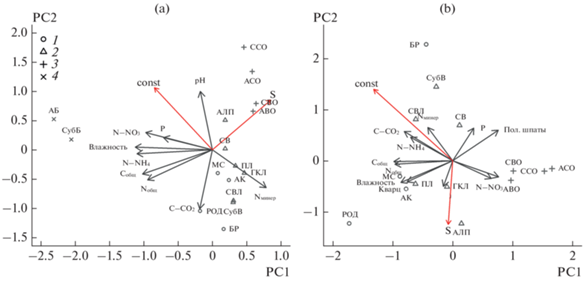
Связи показателя стабилизации и скорости разложения со свойствами почв. В четырех фитоценозах, где STBI и kTBI были измерены дважды в разные годы, значения STBI не различались по годам, однако kTBI в 2015–2016 гг. была значимо ниже в почвах АЛП, АБ и СубБ (табл. 4).
Таблица 4.
Показатель стабилизации (STBI) и скорость разложения (kTBI, мг/(г сут)) в высокогорных растительных сообществах, расположенных по высотному градиенту. Названия сообществ см. табл. 1. n – повторность. Если n < 5, чайные пакетики были повреждены во время инкубации. Уровень значимости p по результатам непараметрического теста Манна–Уитни приведен для скорости разложения в сообществах, где измерения были проведены в течение двух сезонов; значимые различия выделены жирным шрифтом. Все различия между значениями S не значимы. Значения S и k для 2011–2012 гг. приведены по [17]
Сообщество | Годы | S | k, мг/(г сут) | |||
---|---|---|---|---|---|---|
n | среднее ± ошибка | n | среднее ± ошибка | p | ||
БР | 2011–2012 | 5 | 0.264 ± 0.016 | 3 | 4.33 ± 0.34 | – |
СВ | 2011–2012 | 5 | 0.267 ± 0.012 | 2 | 4.25 | – |
СубБ | 2011–2012 | 5 | –0.047 ± 0.008 | 5 | 3.38 ± 0.28 | 0.012 |
2015–2016 | 4 | –0.126 ± 0.007 | 5 | 2.44 ± 0.13 | ||
СубВ | 2011–2012 | 5 | 0.257 ± 0.021 | 5 | 4.74 ± 0.26 | – |
СВЛ | 2011–2012 | 5 | 0.267 ± 0.011 | 4 | 4.43 ± 0.31 | – |
МС | 2011–2012 | 5 | 0.360 ± 0.016 | 5 | 4.68 ± 0.41 | – |
ПЛ | 2011–2012 | 5 | 0.324 ± 0.012 | 5 | 3.74 ± 0.39 | – |
РОД | 2011–2012 | 4 | 0.311 ± 0.014 | 5 | 3.54 ± 0.21 | – |
ГКЛ | 2011–2012 | 5 | 0.252 ± 0.015 | 5 | 3.78 ± 0.37 | 0.529 |
2015–2016 | 5 | 0.278 ± 0.015 | 5 | 3.54 ± 0.34 | ||
АЛП | 2011–2012 | 5 | 0.363 ± 0.014 | 5 | 4.52 ± 0.34 | 0.046 |
2015–2016 | 5 | 0.366 ± 0.021 | 5 | 3.30 ± 0.35 | ||
АК | 2011–2012 | 5 | 0.359 ± 0.014 | 5 | 3.72 ± 0.22 | – |
АВО | 2015–2016 | 5 | 0.355 ± 0.014 | 5 | 2.14 ± 0.09 | – |
АБ | 2011–2012 | 5 | 0.317 ± 0.020 | 5 | 4.66 ± 0.17 | 0.012 |
2015–2016 | 5 | 0.279 ± 0.014 | 5 | 3.24 ± 0.17 | ||
АСО | 2015–2016 | 5 | 0.237 ± 0.013 | 4 | 3.48 ± 0.38 | – |
ССО | 2015–2016 | 5 | 0.294 ± 0.013 | 5 | 2.94 ± 0.13 | – |
СВО | 2015–2016 | 5 | 0.412 ± 0.028 | 5 | 2.40 ± 0.13 | – |
Содержание ПОВ оказалось значимым предиктором для показателя стабилизации (Cмикр – 52.1% дисперсии, p < 0.001; Cорг-экстр – 33.3%, p = = 0.012; ППП – 26.3%, p = 0.016; Собщ – 24.9%, p = = 0.026), но без учета болотных сообществ эта связь не выявлялась. Скорость разложения была значимо связана только с Собщ (14.1% дисперсии, p = 0.033).
Лучшая модель для STBI включала C : Nорг-экстр и абсолютную высоту, вместе они объясняли 72.8% дисперсии. Стабилизация возрастала с высотой и уменьшалась при более высоком отношении C : Nорг-экстр (табл. 5). Однако при исключении болот (СубБ и АБ) из анализа, ни один предиктор не был значим для STBI. Таким образом, на расширенной группе сообществ, включая осыпи, подтверждены ранее отмеченные связи STBI с высотой [17]. Снижение STBI с повышением температуры было значимым только в почвах травяных сообществ и болот, но не под древесной растительностью в Италии [42]. Также известно, что показатель стабилизации и скорость разложения сильнее зависят от температуры во влажных местообитаниях, чем в сухих [52], что объясняет незначимость фактора высоты в моделях, где были исключены болотные сообщества. Кроме того, отмечена отрицательная корреляция STBI с общим количеством азота на горных лугах Швейцарии и Австрии [8]. В настоящем случае связь STBI с Nобщ была не значимой, но отмечены отрицательные связи с N–NH4 (p = 0.003) и Nорг-экстр (p = 0.024).
Таблица 5.
Результаты моделей множественной регрессии, связывающих показатель стабилизации STBI и kTBI (зависимые переменные) с факторами среды, а также моделей, связывающих потери при прокаливании (ППП, %) и содержание углерода (Cобщ, %) с показателем стабилизации STBI, скоростью разложения kTBI и приростом корней. n – число сообществ, включенных в модель. R2m – коэффициент аппроксимации только для фиксированных факторов. Эффект: – отрицательный, + положительный. * – p < 0.05, ** – p < 0.01, *** – p < 0.001, n.s. – связь не значима. Df – число степеней свободы. p – уровень значимости
Зависимые переменные | n | R2m | Предикторы | Параметры моделей | |||
---|---|---|---|---|---|---|---|
эффект | χ2 | Df | p | ||||
Все сообщества | |||||||
STBI | 16 | 0.728 | C : Nорг-экстр | – | 35.19 | 1 | <0.001*** |
Высота | + | 9.78 | 1 | 0.002** | |||
kTBI | 16 | 0.250 | C : Nорг-экстр/C : Nобщ | – | 14.48 | 1 | <0.001*** |
ППП | 16 | 0.396 | kTBI | + | 6.09 | 1 | 0.013* |
STBI | – | 4.82 | 1 | 0.028* | |||
Cобщ | 16 | 0.406 | Прирост корней, kTBI | + | 5.68 | 1 | 0.017* |
+ | 7.14 | 1 | 0.007** | ||||
Без болотных сообществ | |||||||
kTBI | 14 | 0.496 | N NH4 | + | 66.09 | 1 | <0.001*** |
Промерзание почвы | + | 9.56 | 1 | 0.002** | |||
ППП | 14 | 0.415 | kTBI | + | 9.98 | 1 | 0.002** |
Cобщ | 14 | 0.385 | kTBI | + | 9.06 | 1 | 0.003** |
Сообщества без древесных доминантов | |||||||
ППП | 12 | 0.666 | Прирост корней | + | 5.15 | 1 | 0.023* |
STBI | – | 4.16 | 1 | 0.041* | |||
kTBI | + | 3.76 | 1 | 0.052n.s. | |||
Cобщ | 12 | 0.537 | Прирост корней | + | 14.31 | 1 | <0.001*** |
Лучшим предиктором для kTBI оказалось отношение C : Nорг-экстр/C : Nобщ (25.0% дисперсии, табл. 5, рис. 2a). При исключении из анализа болот лучшая модель объясняла 49.6% дисперсии и включала содержание аммонийного азота и промерзание почвы. В автоморфных почвах kTBI повышалась при увеличении N–NH4 и на промерзающих почвах. Тем не менее отрицательная связь между kTBI и C : Nорг-экстр/C : Nобщ сохранялась и в автоморфных почвах (R2 = 0.258, p < 0.001).
Рис. 2.
Связь между константой скорости разложения kTBI и степенью насыщенности экстрагируемой фракции органического вещества азотом (a) и связь между степенью насыщенности экстрагируемой фракции органического вещества азотом и базальным дыханием (БД, C–CO2 мг/(кг ч)) в высокогорных сообществах Тебердинского национального парка. Линии регрессии и доверительные интервалы для отдельных факторов, коэффициенты детерминации (R2) и значения p основаны на простой линейной регрессии средних значений. Сокращения названий сообществ см. табл. 1. Почвы: 1 – литоземы темногумусовые; 2 – литоземы перегнойно-темногумусовые и литозем грубогумусовый; 3 – петроземы гумусовые; 4 – торфяно-литоземы.
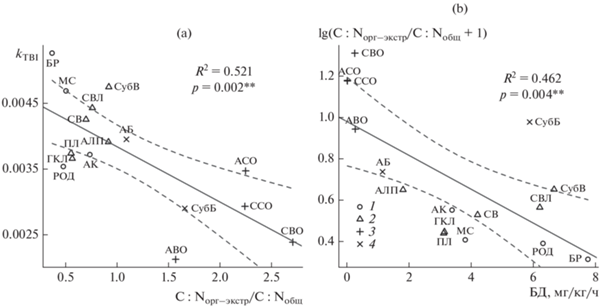
Экстрагируемая фракция ПОВ субнивальных и альпийских осыпей характеризуется довольно высокой степенью насыщения азотом C : Nорг-экстр = = 12.8–14.8. При улучшении гидротермических условий для развития растений и микроорганизмов соотношение C : Nорг-экстр снижается до 7.0–13.4 в горизонтах AU и AH литоземов. При замедлении микробиологического разложения насыщение ПОВ азотом горизонтов Т торфяных почв заметно снижается C : Nорг-экстр = 20.4–39.5. Необходимо отметить, что в почвах, где активность микроорганизмов замедлена (на осыпях – низкими температурами, на болотах – дефицитом кислорода) C : Nорг-экстр больше или равно C : Nобщ. В случае активной деструкции растительного материала в почвах под луговой и лесной растительностью степень насыщения ПОВ азотом экстрагируемой фракции, напротив, меньше (рис. 2b), что подтверждается связью kTBI и C : Nорг-экстр/C : Nобщ. Микробная минерализация ПОВ в автоморфных почвах в значительной степени определяется климатическими условиями. Так, базальное дыхание почвы объясняло 28.5% дисперсии kTBI (p < 0.001). БД возрастало с уменьшением абсолютной высоты от 0–0.3 на осыпях до 1.8 мг С–СО2/(г ч) на АЛП, далее до 3.1–3.8 мг С–СО2/(г ч) альпийских луговых сообществах и МС и до 6.2–7.8 мг С–СО2/(г ч) в почвах субальпийских луговых и лесных сообществ. В болотных почвах БД лимитировано качеством органического субстрата. Так, дыхание олиготрофного альпийского болота значительно ниже, чем мезотрофного субальпийского (1.2 и 5.9 мг С–СО2/(г ч) соответственно).
Интенсивность минерализации азота определяется в первую очередь качеством субстрата, поэтому в почвах с дефицитом азота для развития микроорганизмов значения Nминер близки к нулю или даже отрицательны (осыпи, АЛП, РС, СВ, а также АБ и СубБ). По всем изученным сообществам Nминер не связана ни с STBI, ни с kTBI, однако в автоморфных почвах Nминер была предиктором kTBI (R2 = 0.162, p = 0.028).
В целом в изученных почвах Тебердинского национального парка стабилизация и разложение определялись разными факторами, что подтверждает выводы работы [52].
Связь содержания ПОВ с STBI, kTBI и продуктивностью корней. Стабилизация и скорость разложения совместно объясняли 42.6% дисперсии ППП, а содержание Cорг было связано со скоростью разложения в сочетании с продуктивностью корней. И ППП, и Cорг увеличивались при высокой скорости разложения (табл. 5), в то же время ППП уменьшались при низких значениях STBI. Это противоречит нашей гипотезе, что для наибольшего содержания ПОВ высокие значения STBI должны сочетаться с низкой kTBI. Показатель стабилизации также был отрицательно скоррелирован с содержанием органического углерода в почвах разных местообитаний Швеции, хотя при совместном рассмотрении образцов из Швеции и Австрии связь оказалась положительной [51]. При исключении из анализа болотных сообществ, значимым предиктором как потерь при прокаливании, так и Cорг, оказалась только kTBI.
За исключением почвы СубБ, диапазон наблюдаемых значений STBI в нашей работе не очень широк, а сами значения достаточно высокие (но не крайне высокие). В то же время kTBI везде намного ниже значений, приведенных в работе [28], и в наших данных нет комбинации низких значений STBI и высоких kTBI. Таким образом, можно бы ожидать высокое содержание ПОВ в почвах под всеми растительными сообществами. Тем не менее, содержание ПОВ в них крайне изменчиво, и, очевидно, другие факторы влияют на его накопление, например продуктивность сообщества и функциональные признаки доминантов. В свою очередь ПОВ может влиять на параметры разложения за счет изменения физико-химических параметров почвы, например влажности [48].
Почвы альпийских и субнивальных осыпей сочетают высокую стабилизацию и низкую скорость разложения, но при этом здесь наименьшее содержание ПОВ. Эти сообщества отличаются низкой продуктивностью: их проективное покрытие обычно не превышает 10% [39], таким образом, здесь аккумуляция ПОВ ограничена поступлением растительных остатков.
Субальпийское болото отличается от других сообществ сочетанием низкой стабилизации с низкой скоростью разложения. Отрицательные значения фактора стабилизации также отмечены для некоторых болот Италии [42]. Снижение стабилизации с увеличением влажности отмечено и в заболоченных местообитаниях приливно-отливной зоны [35]. Низкие значения STBI в таких условиях могут быть связаны с быстрой потерей массы зеленым чаем за счет вымывания экстрагируемой фракции ОВ при влажности свыше 25% [7]. Низкая стабилизация на изученном субальпийском болоте может быть также связана с величиной pH около 6, что подавляет включение ПОВ в органо-минеральные соединения [49], несмотря на высокое по сравнению с другими изученными сообществами (287 ммоль(+)/кг) содержание кальция [33]. В целом, болота отличаются относительно низкой скоростью разложения из-за высокой обводненности и анаэробной среды, которая неблагоприятна для микробной активности [46]. Тем не менее, связь между kTBI и содержанием воды была не значимой в высокогорных сообществах. Высокое содержание ПОВ на болотах также может быть связано со свойствами растений, так как ветошь мхов, которые обильны в этих сообществах, устойчива к разложению и разлагается медленнее, чем ветошь лишайников и сосудистых растений [24, 31].
В двух сообществах с высоким участием Rhododendron caucasicum, накопление ПОВ может быть связано с относительно высоким поступлением трудноразлагаемого опада этого вида [18].
В качестве показателя продуктивности альпийских фитоценозов использовали величину прироста тонких корней. При включении всех сообществ в модель зависимости ПОВ от параметров разложения стандартного материала и продуктивности продуктивность корней была значима для Cорг, но была исключена в процессе автоматической пошаговой регрессии для ППП. Однако, когда рассматривали только сообщества с травянистыми доминантами, ППП также увеличивались с продуктивностью корней (рис. 3b), в то время как отрицательная связь с STBI и отсутствие значимой связи с kTBI сохранились (табл. 6 ).
Рис. 3.
Связь потери органического вещества при прокаливании (ППП) и содержания углерода с биомассой прироста тонких корней (на 47.7 см3 за 60 дней). Линии регрессии и доверительные интервалы для отдельных факторов, коэффициенты детерминации (R2) и значения p основаны на простой линейной регрессии средних значений только для сообществ без древесных доминантов. Сокращения названий сообществ см. табл. 1. Почвы: 1 – литоземы темногумусовые; 2 – литоземы перегнойно-темногумусовые и литозем грубогумусовый; 3 – петроземы гумусовые; 4 – торфяно-литоземы.
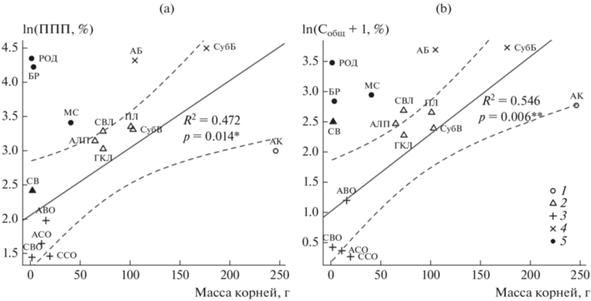
Положительная связь между ПОВ и продукцией корней для травяных сообществ подчеркивает роль продуктивности в накоплении ПОВ. Сведения о роли корней в динамике ПОВ до сих пор противоречивы [13]: они могут одновременно стимулировать как стабилизацию органического вещества, так и его разрушение (прайминг-эффект), и баланс между этими процессами зависит от условий конкретного местообитания. Помимо общего поступления растительных остатков в почву, биомасса тонких корней и скорость их круговорота регулирует микробную активность различными способами, включая конкуренцию за элементы минерального питания и предпочтительное потребление микроорганизмами углерода из корневых выделений, что проявляется в уменьшении скорости разложения ПОВ [11]. Однако это может быть справедливо только для сообществ с доминированием арбускулярной микоризы, в то время как при наличии эктомикоризных грибов добавление опада усиливает разложение ПОВ [26], и это частично может объяснить низкое содержание ПОВ в почве сосняка вейникового. Таким образом, в изученных альпийских сообществах прайминг-эффект может быть слабо выражен в связи с неблагоприятными условиями для активной деятельности микроорганизмов. От зависимости между ПОВ и продукцией корней отклоняются альпийские ковры. На них отмечена самая высокая среди изученных сообществ продукция корней, что, вероятно, является адаптацией к короткому вегетационному периоду. Положительная связь между ПОВ и приростом корней может быть также результатом связей этих переменных с содержанием воды в почве. Усиленный рост корней при высоком объемном содержании влаги отмечен для высокогорных почв Тебердинского национального парка [41], а увеличение содержания ПОВ ведет к росту влагоемкости в большинстве почв [48].
ЗАКЛЮЧЕНИЕ
Почвы субальпийских, альпийских и субнивальных растительных сообществ Северо-Западного Кавказа отличаются высоким разнообразием по содержанию ПОВ и параметрам разложения в них стандартного органического материала. Ведущими градиентами в изученных почвах являются содержание влаги (автоморфные – гидроморфные почвы) и сопутствующее накопление ПОВ, а также высотный градиент, скоррелированный с базальным дыханием почвы. Выявлены неожиданные связи между содержанием ПОВ и ранними стадиями разложения стандартного материала: для богатых органическим веществом почв характерны низкий показатель стабилизации и относительно высокая скорость разложения. Положительная связь скорости прироста тонких корней с содержанием ПОВ свидетельствует о важной роли продуктивности для накопления органического вещества в почвах альпийских сообществ северо-западного Кавказа.
Список литературы
Вертелина О.С., Онипченко В.Г., Макаров М.И. Первичные минералы и процессы выветривания в высокогорных почвах Тебердинского заповедника // Вестник Моск. ун-та. Сер. 17, почвоведение. 1996. № 1. С. 3–10.
Классификация и диагностика почв России. Смоленск: Ойкумена, 2004. 342 с.
Макаров М.И., Волков А.В., Малышева Т.И., Онипченко В.Г. Фосфор, азот и углерод в почвах субальпийского и альпийского поясов Тебердинского заповедника // Почвоведение. 2001. № 1. С. 62–71.
Семенов В.М., Тулина А.С., Семенова Н.А., Иванникова Л.А. Гумификационные и негумификационные пути стабилизации органического вещества в почве (обзор) // Почвоведение. 2013. № 4. С. 393–407. https://doi.org/10.7868/S0032180X13040114
Bartoń K. MuMIn: Multi-Model Inference. R package version 1.40.0. 2017. https://CRAN.R-project.org/package=MuMIn
Basile-Doelsch I., Balesdent J., Pellerin S. The mechanisms underlying carbon storage in soil // Biogeosciences. 2020. V. 17. P. 5223–5242. https://doi.org/10.5194/bg-17-5223-2020
Blume-Werry G., Di Maurizio V., Beil I., Lett S., Schwieger S., Kreyling J. Don’t drink it, bury it: comparing decomposition rates with the tea bag index is possible without prior leaching // Plant and Soil. 2021. V. 465. P. 613–621. https://doi.org/10.1007/s11104-021-04968-z
Bohner A., Karrer J., Walcher R., Brandl D., Michel K., Arnberger A., Frank T., Zaller J.G. Ecological responses of semi-natural grasslands to abandonment: case studies in three mountain regions in the Eastern Alps // Folia Geobotanica. 2019. V. 54. P. 211–225. https://doi.org/10.1007/s12224-019-09355-2
Chen L.-F., He Z.-B., Du J., Yang J.-J., Zhu X. Patterns and environmental controls of soil organic carbon and total nitrogen in alpine ecosystems of northwestern China // Catena. 2016. V. 137. P. 37–43. https://doi.org/10.1016/j.catena.2015.08.017
Cheng L., Chen W., Adams T., Wei X., Li L., Mccormack M.L., Deforest J.L., Koide R.T., Eissenstat D.M. Mycorrhizal fungi and roots are complementary in foraging within nutrient patches // Ecology. 2016. V. 97. P. 2815–2823. https://doi.org/10.1002/ecy.1514
Cheng W., Kuzyakov Y. Root effects on soil organic matter decomposition / Roots and Soil Management: Interactions between Roots and the Soil // Agronomy Monograph. 2005. V. 48. P. 119–143.
Davidson E.A., Janssens I.A. Temperature sensitivity of soil carbon decomposition and feedbacks to climate change // Nature. 2006. V. 440. P. 165–173. https://doi.org/10.1038/nature04514
Dijkstra F.A., Zhu B., Cheng W. Root effects on soil organic carbon: a double-edged sword // New Phytologist. 2021. V. 230. P. 60–65.
Djukic I., Zehetner F., Tatzber M., Gerzabek M.H. Soil organic-matter stocks and characteristics along an Alpine elevation gradient // J. Plant Nutr. Soil Sci. 2010. V. 173. P. 30–38. https://doi.org/10.1002/jpln.200900027
Dorich R.A., Nelson D.W. Evaluation of manual cadmium reduction methods for determination of nitrate in potassium chloride extracts of soils // Soil Sci. Soc. Am. J. 1984. V. 48. P. 72‒75.
Egli M., Sartori G., Mirabella A., Favilli F., Giaccai D., Delbos E. Effect of north and south exposure on organic matter in high Alpine soils // Geoderma. 2009. V. 149. P. 124–136. https://doi.org/10.1016/j.geoderma.2008.11.027
Elumeeva T.G., Onipchenko V.G., Akhmetzhanova A.A., Makarov M.I., Keuskamp J.A. Stabilization versus decomposition in alpine ecosystems of the Northwestern Caucasus: the results of a tea bag burial experiment // J. Mount. Sci. 2018. V. 15. P. 1633–1641. https://doi.org/10.1007/s11629-018-4960-z
Elumeeva T.G., Onipchenko V.G., Cornelissen J.H.C., Semenova G.V., Perevedentseva L.G., Freschet G.T., van Logtestijn R.S.P., Soudzilovskaia N.A. Is intensity of plant root mycorrhizal colonization a good proxy for plant growth rate, dominance and decomposition in nutrient poor conditions // J. Veg. Sci. 2018b. V. 29. P. 715–725. https://doi.org/10.1111/jvs.12651
Fanin N., Bezaud S., Sarneel J.M., Cecchini S., Nicolas M., Augusto L. Relative importance of climate, soil and plant functional traits during the early decomposition stage of standardized litter // Ecosystems. 2020. V. 23. P. 1004–1007. https://doi.org/10.1007/s10021-019-00452-z
Fontaine S., Mariotti A., Abbadie L. The priming effect of organic matter: a question of microbial competition? // Soil Biol. Biochem. 2003. V. 35. P. 837–843.
Fox J., Weisberg S. An {R} Companion to Applied Regression, Third Edition. Thousand Oaks CA: Sage. 2019. https://socserv.socsci.mcmaster.ca/jfox/Books/Companion
Freschet G.T., Cornwell W.K., Wardle D.A., Elumeeva T.G., Liu W., Jackson B.G., Onipchenko V.G., Soudzilovskaia N.A., Tao J., Cornelissen J.H.C. Linking litter decomposition of above- and below-ground organs to plant-soil feedbacks worldwide // J. Ecol. 2013. V. 101. P. 943–952. https://doi.org/10.1111/1365-2745.12092
Ghabbour E.A., Davies G., Cuozzo N.P., Miller R.O. Optimized conditions for determination of total soil organic matter in diverse samples by mass loss on ignition // J. Plant Nutr. Soil Sci. 2014. V. 177. P. 914–919. https://doi.org/10.1002/jpln.201400326
Hobbie S.E. Temperature and plant species control over litter decomposition in Alaskan tundra // Ecol. Monographs. 1996. V. 66. P. 503–522.
Hoffmann U., Hoffmann T., Jurasinski G., Glatzel S., Kuhn N.J. Assessing the spatial variability of soil organic carbon stocks in an alpine setting (Grindelwald, Swiss Alps) // Geoderma. 2014. V. 232–234. P. 270–283. https://doi.org/10.1016/j.geoderma.2014.04.038
Jacobs L.M., Sulman B.N., Brzostek E.R., Feighery J.J., Philips R.P. Interactions among decaying leaf litter, root litter and soil organic matter vary with mycorrhizal type // J. Ecol. 2018. V. 106. P. 502–513. https://doi.org/10.1111/1365-2745.12921
Kandeler E. Ammonium // Methods in Soil Biology. Berlin, Heidelberg: Springer-Verlag, 1996. P. 406‒408.
Keuskamp J.A., Dingemans B.J.J., Lehtinen T., Sarneel J.M., Hefting M.M. Tea Bag Index: a novel approach to collect uniform decomposition data across ecosystems // Methods Ecol. Evol. 2013. V. 4. P. 1070–1075. https://doi.org/10.1111/2041-210X.12097
Kirschbaum M.U.F. The temperature dependence of soil organic matter decomposition, and the effect of global warming on soil organic storage // Soil Biol. Biochem. 1995. V. 27. P. 753–760. https://doi.org/10.1016/0038-0717(94)00242-S
Kopáček J., Kaňa J., Šantrůčková H. Pools and composition of soils in the alpine zone of the Tatra Mountains // Biologia, Bratislava. 2006. V. 61. Suppl. 18. P. 35–49. https://doi.org/10.2478/s11756-006-0118-5
Lang S.I., Cornelissen J.H.C., Klahn T., van Logtestijn R.S.P., Broekman R., Schweikert W., Aerts R. An experimental comparison of chemical traits and litter decomposition rates in a diverse range of subarctic bryophyte, lichen and vascular plant species // J. Ecol. 2009. V. 97. P. 886–900. https://doi.org/10.1111/j.1365-2745.2009.01538.x
Lehmann J., Hansel C.M., Kaiser C., Kleber M., Maher K., Manzoni S., Nunan N., Reichstein M. et al. Persistence of soil organic carbon caused by functional complexity // Nature Geoscience. 2020. V. 13. P. 529–534. https://doi.org/10.1038/s41561-020-0612-3
Malysheva T.I., Onipchenko V.G., Makarov M.I., Volkov A.V., Bulatnikova I.V. Soils and nutrient turnover // Alpine ecosystems in the Northwestern Caucasus. Dordrecht: Kluwer, 2004. P. 9–24.
Moar S.E.L., Wilson S.D. Root responses to nutrient patches in grassland and forest // Plant Ecol 2006. V. 184. P. 157–162. https://doi.org/10.1007/s11258-005-9059-2
Mueller P., Schile-Beers L.M., Mozdzer T.J., Chmura G.L., Dinter T., Kuzyakov Y., de Groot A.V., Esselink P. et al. Global-change effects on early-stage decomposition processes in tidal wetlands – implications from a global survey using standardized litter // Biogeosciences. 2018. V. 15. P. 3189–3202.
Nakagawa S., Schielzeth H. A general and simple method for obtaining R2 from generalized linear mixed-effects models // Methods Ecol. Evol. 2013. V. 4. P. 133–142. https://doi.org/10.1111/j.2041-210x.2012.00261.x
Ohtsuka T., Hirota M., Zhang X., Shimono A., Senga Y., Du M., Yonemura S., Kawashima S., Tang Y. Soil organic carbon pools in alpine to nival zones along an altitudinal gradient (4400–5300 m) on the Tibetan Plateau // Polar Sci. 2008. V. 2. P. 277–285. https://doi.org/10.1016/j.polar.2008.08.003
Oksanen J., Blanchet F.G., Friendly M., Kindt R., Legendre P., McGlinn D., Minchin P.R., O’Hara R.B., Simpson G.L., Solymos P., Stevens M.H.H., Szoecs E., Wagner H. vegan: Community Ecology Package. R package version 2.5-7. 2020. https://CRAN.R-project.org/package=vegan
Onipchenko V.G. Alpine vegetation of the Teberda Reserve, the Northwestern Caucasus. Veröffentlichungen des Geobotanischen Institutes der ETH. Heft 130. Zürich: Stiftung Rübel, 2002.
Onipchenko V.G. Geography, geology, climate and the communities studied // Alpine Ecosystems in the Northwestern Caucasus. Dordrecht: Kluwer, 2004. P. 1–8.
Onipchenko V.G., Gulov D.M., Ishbirdin A.R., Makarov M.I., Akhmetzhanova A.A., Logvinenko O.A., Khubieva O.P., Tekeev D.K., Elumeeva T.G. Analysis of fine root production features in high mountain communities by ingrowth method using filter balls // Contemporary Problems of Ecology. 2021. V. 14. P. 456–464. https://doi.org/10.1134/S1995425521050085
Petraglia A., Cacciatori C., Chelli S., Fenu G., Calderisi G., Gargano D., Abeli T., Orsenigo S., Carboniani M. Litter decomposition: effects of temperature driven by soil moisture and vegetation type // Plant Soil. 2019. V. 435. P. 187–200. https://doi.org/10.1007/s11104-018-3889-x
Pinheiro J., Bates D., DebRoy S., Sarkar D., R Core Team. nlme: Linear and Nonlinear Mixed Effects Models. R package version 3.1-152. 2021. http://CRAN.R-project.org/package=nlme
Pierson D., Evans L., Kayhani K., Bowden R.D., Nadelhoffer K., Simpson M., Laitha K. Mineral stabilization of soil carbon is suppressed by live roots, outweighting influences from litter quality or quantity // Biogeochem. Lett. 2021. V. 154. P. 433–449. https://doi.org/10.1007/s10533-021-00804-9
Poirier V., Roumet C., Munson A.D. The root of matter: linking root traits and soil organic matter stabilization processes // Soil Biol. Biochem. 2018. V. 120. P. 246–259. https://doi.org/10.1016/j.soilbio.2018.02.016
Prescott C.E. Litter decomposition: what controls it and how we can alter it to sequester more carbon to forest soils? // Biogeochemistry. 2010. V. 101. P. 133–149. https://doi.org/10.1007/s10533-010-9439-0
Pohler T. The Pairwise Multiple Comparison of Mean Ranks Package (PMCMR). R package. 2014. http://CRAN.R-project.org/package=PMCMR.
Rawls W.J., Pachepsky Y.A., Ritchie J.C., Sobecki T.M., Bloodworth H. Effect of soil organic carbon on soil water retention // Geoderma. 2003. V. 116. P. 61–76. https://doi.org/10.1016/S0016-7061(03)00094-6
Rowley M.C., Grand S., Verrecchia E. Calcium-mediated stabilization of soil organic carbon // Biogeochemistry. 2018. V. 137. P. 27–49. https://doi.org/10.1007/s10533-017-0410-1
R Core Team. R: A language and environment for statistical computing. R Foundation for Statistical Computing, Vienna, Austria. 2021. https://www.R-project.org/.
Sandén T., Spiegel H., Wenng H., Schwarz M., Sarneel J.M. Learning science during teatime: Using a citizen science approach to collect data on litter decomposition in Sweden and Austria // Sustainability. 2020. V. 12. P. 7745. https://doi.org/10.3390/su12187745
Sarneel J., Sundkvist M.K., Molau U., Björkman M.P., Alatalo J.M. Decomposition rate and stabilization across six tundra vegetation types exposed to >20 years of warming // Sci. Total Environ. 2020. V. 724. P. 138304. https://doi.org/10.1016/j.scitotenv.2020.138304
Schmidt M.W.I., Torn M.S., Abiven S., Dittmar T., Guggenberger G., Janssens I.A., Kleber M., Kögel-Knabner I., Lehmann J., Manning D.A., Nannipieri P., Rasse D.P., Weiner S., Trumbore S.E. Persistence of soil organic matter as an ecosystem property // Nature. 2011. V. 478. P. 49–56.
Six J., Conant R.T., Paul E.A., Paustian K. Stabilization mechanisms of soil organic matter: Implications for C-saturation of soils // Plant Soil. 2002. V. 241. P. 155–176. https://doi.org/10.1023/A:1016125726789
Sørensen M.V., Strimbeck R., Nystuen K.O., Kapas R.E., Enquist B.J., Graae B.J. Draining the pool? Carbon storage and fluxes in three alpine plant communities // Ecosystems. 2018. V. 21. P. 316–330. https://doi.org/10.1007/s10021-017-0158-4
Stockmann U., Adams M.A., Crawford J.W., Field D.J., Henakaarchchi N., Jenkins M., Minasny B., McBratney A.B. et al. The knowns, known unknowns and unknowns of sequestration of soil organic carbon // Agriculture Ecosys. Envir. 2013. V. 164. P. 80–99. https://doi.org/10.1016/j.agee.2012.10.001
Veen G.F., Freschet G.T., Ordonez A., Wardle D. Litter quality and environmental controls of home-field advantage effects on litter decomposition // Oikos. 2015. V. 124. P. 187–195.
Venn S.E., Thomas H.J.D. Snowmelt timing affects short-term decomposition rates in an alpine snowbed // Ecosphere. 2021. V. 12. № 3. P. e03393. https://doi.org/10.1002/ecs2.3393
Дополнительные материалы отсутствуют.