Коллоидный журнал, 2023, T. 85, № 4, стр. 443-452
Бикомпонентные серебросодержащие наночастицы: связь морфологии и электрокинетического потенциала
М. И. Лернер 1, 2, О. В. Бакина 1, *, С. О. Казанцев 1, Е. А. Глазкова 1, Н. В. Сваровская 1
1 Институт физики прочности и материаловедения Сибирского отделения
Российской академии наук
634021 Томск, пр. Академический 2/4, Россия
2 Севастопольский государственный университет
299053 Севастополь,
ул. Университетская, 33, Россия
* E-mail: ovbakina@ispms.tsc.ru
Поступила в редакцию 29.04.2023
После доработки 24.05.2023
Принята к публикации 24.05.2023
- EDN: OQBZFC
- DOI: 10.31857/S002329122360030X
Аннотация
В настоящей работе исследованы химический состав, структура и электрокинетические характеристики содержащих серебро наночастиц ZnO/Ag с морфологией янус-наночастиц, гетерофазных наночастиц Cu/Ag с равномерным распределением компонентов в частице и наночастиц TiO2/Ag с поверхностью, декорированной Ag. Проанализировано влияние структуры поверхности на положение изоэлектрической точки и величины электрокинетического потенциала для образцов наночастиц, полученных совместным электрическим взрывом двух проводников. Изучено влияние расположения серебра на электрокинетические характеристики наночастиц и проведено сравнение данных характеристик с механическими смесями, взятыми в том же массовом соотношении.
ВВЕДЕНИЕ
В настоящее время чрезмерное применение антибиотиков приводит к появлению устойчивых бактериальных штаммов, вызывающих осложнения при течении инфекционных заболеваний [1]. Анализ проведенных исследований [2] показал, что необходимы новые подходы к разработке следующего поколения антимикробных препаратов. В связи с этим активно ведутся работы по получению альтернативных антимикробных агентов широкого спектра действия, таких как наночастицы (НЧ) металлов и оксидов металлов, включая бикомпонентные НЧ [3].
Известно, что НЧ благородных металлов, таких как серебро и золото, проявляют антибактериальное действие в отношении широкого спектра микроорганизмов, в связи с чем они используются в пищевых консервантах, стоматологических полимерных композитах, косметике, покрытиях медицинских устройств и медицинских инструментов, имплантатах [4]. Несмотря на явные доказательства антибактериальной эффективности серебра, вызывает опасения цитотоксичность серебра в действующих концентрациях [5]. Кроме того, бактериальная устойчивость к катионному серебру (Ag+) известна уже много лет, но недавно было обнаружено, что она также возможна к НЧ Ag [6]. В связи с этим перспективным является применение бикомпонентных НЧ [3].
Бикомпонентные наночастицы, имея особую структуру, отличную от монометаллических наночастиц, могут обладать свойствами, отличными от свойств отдельных металлов и оксидов металлов [7]. Возможность получения наночастиц с контролируемой морфологией позволяет расширить области их применения в биологии и медицине [8]. До недавнего времени большинство опубликованных работ были сконцентрированы на синтезе наночастиц сплавов. В наши дни исследователи сосредоточили свое внимание на селективной подготовке новых бикомпонентных наночастиц с различной структурой и сложной архитектурой, потому что их специфическая геометрия приводит к необычным физическим и химическим свойствам, которые могут быть использованы при разработке перспективных наноструктурированных материалов. Бикомпонентные НЧ образуются путем объединения двух разных типов НЧ и могут иметь разнообразную морфологию и структуру [9]. Обычно они проявляют более интересные свойства по сравнению с соответствующими монометаллическими НЧ, что объясняется синергетическими свойствами двух разных металлических частей. Настройка свойств и производительности может быть достигнута путем выбора правильной комбинации компонентов. Бóльшая часть публикуемых работ посвящена получению бикомпонентных НЧ с антимикробными свойствами, в которых серебро выступает в качестве ядра или оболочки частицы. Так, НЧ Ag/Au с ядром из золота, на котором осаждались частицы серебра, обладали повышенной активностью как по отношению к грамположительным, так и грамотрицательным бактериям [10]. Синтезированы бикомпонентные структуры AgCo [11], AgZn [12], AgFe [13] и исследована их антимикробная активность. Для бикомпонентных НЧ со структурой ядро‒оболочка Ag/Cu, полученных методом химического осаждения из раствора, отмечено снижение токсичности по сравнению с наночастицами серебра и увеличение антибактериальной активности по сравнению с отдельными частицами меди и серебра [14].
Как показано в большом количестве работ, обобщенных в обзоре [15], ключевую роль во взаимодействии наночастиц с микроорганизмами играет электростатическое взаимодействие между ними. Поверхность бактериальной клетки заряжена отрицательно благодаря наличию карбоксильных групп сиаловых или тейхоевых кислот, которые незначительно нейтрализуются положительно заряженными четвертичными аммониевыми группами мембранных фосфолипидов [16]. Для более эффективного взаимодействия с бактериальной стенкой НЧ должны иметь положительный заряд при физиологических значениях рН 7.0‒7.5 [17]. Так, модификация поверхности НЧ Au катионными лигандами способствовала адгезии наночастиц к поверхности бактерий [18]. Авторы [19] модифицировали поверхность наночастиц Au лигандами с различным зарядом и ориентацией. Установлено, что НЧ с катионным зарядом во внешнем слое проявляли более высокую противомикробную активность по сравнению с частицами, имеющими положительно заряженное ядро и отрицательно заряженную оболочку.
Наиболее перспективными для получения бикомпонентных НЧ являются физические методы с высокими скоростями охлаждения дисперсной фазы: газофазный метод [20], лазерная абляция [21] и электрический взрыв проволочек (ЭВП) [22]. Такие методы позволяют получать наночастицы в количествах, достаточных для промышленного применения, легко масштабируются и позволяют получать нанопорошки требуемого химического и дисперсного состава. ЭВП имеет ряд преимуществ перед другими физическими методами, такие как высокая эффективность передачи энергии, возможность варьирования параметров процесса и, соответственно, свойств порошков, сравнительно узкая функция распределения наночастиц по размерам, низкая стоимость и простота оборудования, а также его небольшой вес и габариты.
В связи с тем, что зарядовые свойства НЧ, в том числе и бикомпонентных, в первую очередь определяются свойствами их поверхности, экспериментальное определение их электрокинетического потенциала представляет несомненный интерес. В настоящей работе с помощью ЭВП синтезированы бикомпонентные серебросодержащие НЧ ZnO/Ag, Cu/Ag, TiO2/Ag с различной локализацией серебра. Проведено исследование и выявлено влияние морфологии НЧ на их зарядовые характеристики – электрокинетический потенциал и точку нулевого заряда. Проведено сравнение определяемых характеристик с механическими смесями наночастиц.
ОБЪЕКТЫ И МЕТОДЫ ИССЛЕДОВАНИЯ
В качестве объектов исследования были выбраны серебросодержащие бикомпонентные НЧ Cu/Ag, TiO2/Ag, ZnO/Ag, полученные совместным электрическим взрывом двух свитых проволок в инертной или кислородсодержащей атмосфере. Детальное описание условий получения наночастиц приведено в табл. 1. Методика и оборудование для получения НЧ металлов и оксидов металлов ЭВП описаны в [23–25]. Непосредственно после синтеза порошки пассивировались кислородом воздуха.
Таблица 1.
Параметры синтеза НЧ электрическим взрывом проволочек
Образец | R, мОм | Среда взрыва | L, мкГн | C, мкФ | U0, кВ | lw, мм | dw, мм |
---|---|---|---|---|---|---|---|
Cu/Ag | 92 | Ar | 74 | 3.2 | 30 | 100 | dCu = 0.30; dAg = 0.36 |
TiO2/Ag | 92 | Ar + O2 | 74 | 2.4 | 27 | 80 | dTi = 0.30; dAg = 0.10 |
ZnO/Ag | 92 | Ar + O2 | 74 | 3.2 | 23 | 90 | dZn = 0.38; dAg = 0.15 |
TiO2 | 92 | Ar + O2 | 74 | 2.4 | 27 | 80 | dTi = 0.40 |
ZnO | 92 | Ar + O2 | 74 | 2.8 | 28 | 100 | dZn = 0.38 |
Ag | 92 | Ar | 74 | 3.2 | 33 | 80 | dAg = 0.25 |
Параметры электрического взрыва подбирались таким образом, чтобы обеспечить однородный нагрев по сечению проволок. Диспергирование металла проволок происходило в режиме “быстрого электрического взрыва” [26], при котором, в основном, формируются наночастицы со средним размером 100 нм и узким распределением частиц по размерам.
Для исследования морфологии (размер и форма) НЧ использовали просвечивающую электронную микроскопию (микроскоп JEM 100 CX II, JEOL, Япония, разрешающая способность 0.1 нм). Для оценки размера частиц и построения функции распределения наночастиц по размерам использовали данные просвечивающей электронной микроскопии. При помощи программного пакета “Обработка УДП порошков” обрабатывалось около 2400 частиц. Обработку проводили до тех пор, пока каждая добавленная частица вносила вклад в функцию распределения. Измеряли размер не менее 1500 частиц. Распределения элементов по частице исследовали при помощи энергодисперсионной рентгеновской спектроскопии (анализатор X‒Max, Oxford Instruments). Для оценки размера агломератов наночастиц использовали метод седиментации частиц под действием центробежной силы в градиенте плотностей (CPS DC 24000, CPS Disc Instruments, США). Для проведения исследования микроструктуры наночастиц использовали метод рентгеноструктурного анализа РСА (XRD‒6000, Shimadzu, Япония) на CuКα-излучении с областью углов поворота гониометра 2θ = 10−90 град. со скоростью 2 град/мин и рентгеновской фотоэлектронной спектроскопии РФЭС (SPECS Surface Nano Analysis GmbH, Германия), для получения спектров использовали немонохроматизированное излучение AlKα (hν = 1486.6 эВ). Электрокинетические характеристики суспензии НЧ исследовали методом доплеровского микроэлектрофореза. Измерения электрофоретической подвижности НЧ были проведены в физиологическом растворе 0.9 мас. % NaCl (рН 7) при концентрации суспензии 2 мг/мл, на анализаторе Zetasizer Nano ZS (Malvern, Великобритания). Величина электрокинетического потенциала была рассчитана автоматически по уравнению Смолуховского [27]. Антибактериальную активность НЧ в отношении бактерий S. aureus ATCC 6538 определяли суспензионным методом посевом на плотную питательную среду и по изменению оптической плотности бактериальной суспензии [28].
РЕЗУЛЬТАТЫ И ИХ ОБСУЖДЕНИЕ
ЭВП, основанный на импульсных процессах с высокими скоростями изменения термодинамических параметров системы, является перспективным методом получения наночастиц сложного химического состава [29]. Единственным ограничением ЭВП-технологии является применение металлической проволоки требуемого диаметра. Одним из основных достоинств ЭВП является относительная стабильность фазового и дисперсного состава электровзрывных нанопорошков в нормальных условиях. Другим достоинством ЭВП является возможность гибкого регулирования параметров процесса и, соответственно, характеристик получаемых нанопорошков. Физические свойства взрываемых металлов, соотношение их температур плавления и плотностей, особенности взаимного растворения компонентов в бинарных системах согласно равновесным диаграммам состояния [30] позволяют прогнозировать преимущественное формирование НЧ определенной морфологии.
Согласно диаграмме состояния [30] системы металлов Cu‒Ag, они являются парой с ограниченной растворимостью. Металлы имеют большую разницу в размерах атомных радиусов (около 25%) и близкие температуры плавления – 1085 и 961.8°C соответственно. Энтальпия смешения для данных металлов положительна и составляет 5 кДж/моль. Такая система относится к системам эвтектического типа с ограниченным растворением компонентов друг в друге, для них характерно отсутствие взаимной растворимости в твердом и жидком состоянии. Серебро в меди растворяется слабо, максимальная растворимость серебра в меди составляет 4.9, меди в серебре ‒ 14.1 мас. % [31]. В результате электрического взрыва металлов Cu и Ag формировались наночастицы со структурой т.н. “наносплава” [32] с равномерным распределением отдельных металлов по частице или т.н. гетерофазные наночастицы. На рис. 1 приведены микрофотография, функция распределения по размерам и данные РСА частиц Cu/Ag. Для оценки размера частиц использовали математическую обработку их электронно-микроскопических изображений. Данный метод наиболее применим для построения функции распределения НЧ по размерам, так как позволяет исключить вклад размера агломератов НЧ. Распределение НЧ по размерам согласуется с логарифмическим нормальным законом распределения. Средний размер частиц не превышает 100 нм. Данные РСА показывают, что в образце присутствуют медь и серебро.
Анализ размеров областей когерентного рассеяния (dокр) НЧ Сu/Ag позволяет утверждать, что структура НЧ близка к поликристаллической (dокр < an). Средний размер НЧ составил an = 81 нм, dокр(Ag) = 12 ± 2 нм, dокр(Cu) = 18 ± 4 нм.
В результате ЭВП цинковой и серебряной проволочек в кислородсодержащей атмосфере были получены янус-наночастицы ZnO–Ag, результаты исследования морфологии и структуры которых приведены на рис. 2.
На основании проведенных исследований можно предположить следующий механизм формирования янус-частиц ZnO‒Ag. При ЭВП, когда температура превышает температуры плавления металлов, Ag и Zn могут бесконечно растворяться друг в друге в инертной атмосфере. В кислородсодержащей атмосфере образование наночастиц может происходить одновременно с окислением цинка. Оксид цинка кристаллизуется при 1975°С, а серебро с более низкой температурой плавления (962°С) вытесняется из объема образующейся частицы, в результате чего происходит разделение компонентов и формирование янус-наночастиц. На дифрактограммах видны отдельные компоненты – Ag и ZnO.
При формировании частиц TiO2/Ag при ЭВП в кислородсодержащей атмосфере наблюдалось четкое разделение компонентов (рис. 3). По данным картирования встречаются участки, обогащенные титаном и серебром. Ag и Ti ограниченно растворимы друг в друге в инертной атмосфере, растворимость Ag в Τi составляет 16.3 ат. % при температуре 1020°С [30]. Низкая растворимость металлов обусловлена большой разницей температур плавления, энтальпия смешения для данных металлов положительная. При совместном взрыве проволочек Ag и Ti в кислородсодержащей атмосфере при 1843°Ϲ происходила кристаллизация TiO2, который вытеснял из объема формирующейся частицы жидкое серебро. В результате наблюдалось разделение компонентов и вытеснение серебра на поверхность сферических частиц TiO2 в виде мелких фрагментов размером 10‒50 нм.
Средний размер наночастиц по данным ПЭМ составил 96 ± 2 нм (рис. 3в). Средний размер кристаллитов серебра, рассчитанный методом Уи-льямсона‒Холла составил 25 нм [33].
Таким образом, методом ЭВП были получены НЧ с морфологией янус-наночастиц ZnO/Ag, гетерофазные наночастицы с равномерным распределением кристаллитов по частице Cu/Ag и декорированные серебром сферические частицы TiO2/Ag.
При исследовании двойного электрического слоя на поверхности бикомпонентных НЧ можно предположить, что он будет иметь более сложную структуру, чем на поверхности однородных частиц и коллоидов. Для бикомпонентных наночастиц неравномерное распределение заряда по поверхности может привести к изменению их подвижности и, соответственно, электрокинетического потенциала, который является одной из основных характеристик, определяющих строение двойного электрического слоя и регулирует устойчивость водных суспензий НЧ, а также характер их физического взаимодействия с другими объектами (полимеры, бактерии и т.д.). Наличие положительного электрокинетического потенциала на поверхности НЧ является одним из основных требований к антибактериальным агентам и обеспечивает их электростатическое взаимодействие с поверхностью бактериальной клетки.
Сопоставление электрокинетических характеристик всех исследованных наночастиц в водной суспензии, представленное на рис. 4 и в табл. 2, показывает, что локализация серебра в НЧ способствует отличию электрокинетических характеристик бикомпонентных НЧ от механических смесей, происходит смещение положения изоэлектрической точки (ИЭТ) в нейтральную область для НЧ Cu/Ag (pHТНЗ = 7.41) и в щелочную область для янус-НЧ ZnO/Ag (pHтнз = 9.25). Стоит отметить, что для декорированных НЧ TiO2/Ag положительные величины электрокинетического потенциала наблюдаются только при рН менее 5.
Рис. 4.
Зависимость электрокинетического потенциала НЧ ZnO/Ag (а), Cu/Ag (б) и TiO2/Ag (в) от pH на фоне 0.9% раствора NаCl.
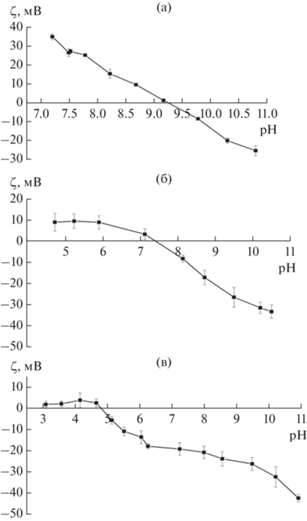
Таблица 2.
Электрокинетические характеристики НЧ и механических смесей компонентов
НЧ | ζ, мВ | рНТНЗ | ζ, мВ |
---|---|---|---|
бикомпонентные НЧ | механические смеси НЧ | ||
Cu/Ag | 4.7 ± 0.2 | 7.41 | –8.1 ± 0.2 |
ZnO/Ag | 35.2 ± 0.7 | 9.25 | 34.3 ± 0.5 |
TiO2/Ag | –19.4 ± 0.4 | 4.80 | –10.75 ± 0.7 |
При исследовании гетерофазных наночастиц Cu/Ag с равномерным распределением компонентов электрокинетический потенциал отличался от значений потенциала для соответствующей механической смеси. Кроме того, полученные данные значительно отличались от отрицательного электрокинетического потенциала НЧ Ag@Cu, полученных химическим осаждением из солей, с различным соотношением компонентов и размером до 15 нм [34]. Он составил 4.7 мВ, что близко к потенциалу нанолистов оксида меди (6.0 мВ), полученных авторами [35]. Это является косвенным доказательством расположения оксида меди на внешней поверхности бикомпонентных наночастиц. При близкой температуре плавления металлов в приповерхностные области частиц твердых растворов в процессе электрического взрыва может вытесняться металл, имеющий меньшую плотность. Медь, имеющая плотность в твердом состоянии 8.93 г/см3 при 960.5°C, вытесняется на поверхность наночастиц из расплава серебра, имеющего плотность 10.49 г/см3. При относительно малом содержании меди она, возможно, образует оболочку наночастицы; при сравнимых соотношениях меди и серебра происходит сегрегация компонентов во всем объеме наночастицы. Однако на кривой распределения элементов по частице участков, обогащенных одним из компонентов, не выявлено (рис. 5).
Для подтверждения наличия меди на поверхности НЧ Cu/Ag проводили дополнительное исследование НЧ методом РФЭС (рис. 6). Как показали данные РФЭС, на поверхности НЧ, помимо металлического серебра, находится медь(II). Присутствие характерного интенсивного “shake-up” сателлита подтверждает нахождение меди в состоянии Cu2+. Как известно, спектр Ag3d представляет собой дублет Ag3d5/2–Ag3d3/2, интегральные интенсивности компонент которого соотносятся как 3 : 2. Величина спин-орбитального расщепления (разница значений энергии связи Ag3d5/2 и Ag3d3/2) составляет 6 эВ. В данном случае спектр Ag3d хорошо описывается одним дублетом с энергией связи Ag3d5/2 в районе 368.0‒368.3 эВ. Значения энергии связи Ag3d5/2 для серебра в различном состоянии изменяются в узком диапазоне 367.5‒368.5 эВ, что затрудняет идентификацию состояния серебра [36]. Для идентификации обычно используют так называемый Оже-параметр α, равный сумме энергии связи Ag3d5/2 и положения максимума Оже-спектра AgMNN на шкале кинетических энергий электронов. Для всех образцов Оже-параметр α находится в диапазоне 725.8‒726.1 эВ, что характерно для серебра в металлическом состоянии. Кроме того, соотношение [Cu]/[Ag] составляет 3.17, что подтверждает обогащение медью поверхности НЧ.
Рис. 6.
Спектры Ag3d и Cu2p образца Cu/Ag. Спектры нормированы на интегральную интенсивность пиков, соответствующих металлам, входящим в состав образцов.
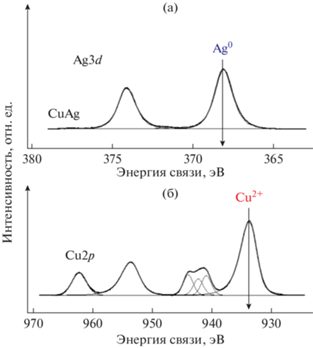
Увеличение содержания серебра в бикомпонентных НЧ Cu/Ag от 20 до 80 мас. % практически не приводило к изменению электрокинетического потенциала суспензии НЧ, он всегда был положительным и близким по значению к потенциалу НЧ CuO (рис. 7).
При измерении электрокинетического потенциала янус-наночастиц ZnO/Ag установлено, что его величина сопоставима со значением для соответствующих механических смесей НЧ (см. табл. 2). Изоэлектрическая точка наночастиц суспензии НЧ ZnO/Ag (рНТНЗ = 9.3), также практически не отличалась от механической смеси НЧ (рНТНЗ = 9.2), взятой в тех же соотношениях. При наложении кривых потенциометрического титрования они практически совпадали (рис. 8).
Рис. 8.
Зависимость электрокинетического потенциала НЧ ZnO/Ag и механической смеси НЧ от pH на фоне 0.9% раствора NаCl.
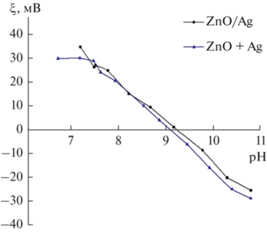
Увеличение содержания серебра в НЧ ZnO/Ag и соответствующих механических смесях приводило к монотонному убыванию электрокинетического потенциала в обоих типах образцов (бикомпонентные наночастицы и механические смеси). Кроме того, величина потенциала оставалась положительной, при этом наблюдалась практически линейная корреляция электрокинетического потенциала с содержанием серебра в образцах (рис. 9). Полученные значения электрокинетического потенциала янус-наночастиц ZnO/Ag значительно отличались от значений для НЧ ZnO/Ag, полученных в других работах химическим осаждением: –13.8 [37], –23 [38], –21 мВ [39], что можно объяснить морфологией наночастиц. В результате химического осаждения образуются частицы преимущественно со структурой ядро–оболочка. Компонент оболочки (серебро) определяет электрокинетический потенциал НЧ. Этот факт подчеркивает перспективы применения ЭВП для получения НЧ ZnO/Ag с положительным электрокинетическим потенциалом.
Измерение электрокинетического потенциала суспензии НЧ TiO2/Ag и сравнение его величины с индивидуальными НЧ TiO2 и Ag сходного размера (рис. 10) показало, что характер изменения потенциала во всем диапазоне pH сходен. С увеличением рН значения электрокинетического потенциала становятся более отрицательными. Величину изоэлектрической точки удалось идентифицировать только для НЧ TiO2 (рНТНЗ = 5.6) и TiO2/Ag (рНТНЗ = 4.8). Значения электрокинетического потенциала НЧ Ag были отрицательными во всем исследованном диапазоне рН. Близкие результаты были получены для коллоидов TiO2 и Ag размером ≈50 нм авторами [40]. Электрокинетический потенциал НЧ TiO2 менее отрицательный, чем у НЧ Ag или НЧ TiO2/Ag. Кривая НЧ TiO2/Ag при рН < 7.1 близка к кривой НЧ Ag, а при рН > 7.1 становится похожей на суперпозицию кривых НЧ TiO2 и Ag. Из этого следует, что при физиологических рН ≈ 7 электрические свойства бикомпонентных НЧ TiO2/Ag определяются Ag на их поверхности. Электрокинетический потенциал НЧ TiO2/Ag более отрицательный, чем соответствующей механической смеси (табл. 1) и механической смеси коллоидных частиц [40], вероятно, за счет экранирования части поверхности TiO2 серебром.
Рис. 10.
Зависимость электрокинетического потенциала НЧ Ag, TiO2/Ag TiO2 от pH на фоне 0.9% раствора NаCl.
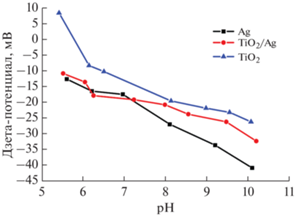
Таким образом, электрокинетический потенциал янус-наночастиц ZnO/Ag (ζ = 35.2 ± 0.7 мВ) является суммой потенциалов каждого компонента. Положительный электрокинетический потенциал гетерофазных НЧ Cu/Ag (ζ = 4.7 ± 0.2 мВ) обусловлен положительно заряженным оксидом меди на поверхности НЧ и экранированием части серебра. Декорированные Ag НЧ TiO2/Ag имеют высокий отрицательный потенциал (ζ = –19.4 ± 0.47 мВ) за счет отрицательного потенциала серебра на поверхности НЧ и экранирования части поверхности более положительно заряженного TiO2.
Согласно литературным данным [41], неспецифическая адгезия бактериальных клеток обусловлена электростатическим взаимодействием бактерий с поверхностями, ожидается, что антимикробная активность НЧ возможно будет коррелировать с их электрокинетическим потенциалом при нейтральном рН. Поскольку бактериальная поверхность обладает отрицательным потенциалом, она будет отталкивать НЧ TiO2/Ag в большей степени. Исследование кинетики роста бактерий S. aureus в физиологическом растворе (рН 7.0) в присутствии синтезированных НЧ в диапазоне концентраций 50–100 мкг/мл показало следующие результаты (рис. 11).
Рис. 11.
Кривые роста бактерий S. aureus в присутствии суспензии НЧ ZnO/Ag (а), Cu/Ag (б), TiO2/Ag (в).
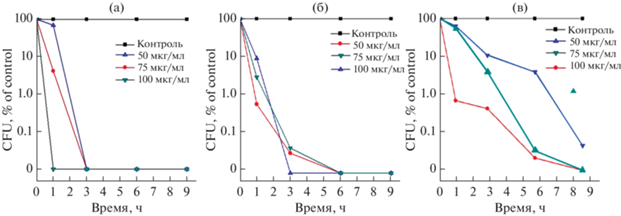
Для всех НЧ значение оптической плотности было ниже, чем в образце контроля (бактерий без НЧ, в растворе 0.9% NaCl), что свидетельствует об антибактериальной активности всех исследованных НЧ. НЧ ZnO/Ag (рис. 11а), обладающие наибольшим положительным электрокинетическим потенциалом, во всех исследованных концентрациях полностью ингибировали рост бактерий через 3 ч экспозиции (через 1 ч в концентрации 100 мкг/мл). Это значение было в 3 раза ниже, чем для частиц Cu/Ag (рис. 11б) и в 9 раз ниже, чем для НЧ TiO2/Ag (рис. 11в). Полученные данные косвенно подтверждают взаимодействие НЧ с поверхностью бактериальной мембраны, а возникающая межфазная разность потенциалов может запускать реакции, приводящие к снижению жизнеспособности бактерий.
ЗАКЛЮЧЕНИЕ
В настоящей работе исследованы состав, структура, электрокинетические и антибактериальные характеристики серебросодержащих бикомпонентных наночастиц с различной морфологией, сформированной в процессе ЭВП. Проанализированы зависимости положения ИЭТ и величин электрокинетического потенциала частиц в зависимости от локализации серебра в частицах. Показано, что гетерофазные НЧ имеют высокий положительный электрокинетический потенциал, янус-наночастицы обладают невысоким положительным электрокинетическим потенциалом, а декорированные серебром наночастицы имеют высокий отрицательный потенциал по сравнению с механическими смесями наночастиц. Установлено, что антибактериальное действие выше у наночастиц с более положительным электрокинетическим потенциалом. Это подтверждает, что механизм антимикробного действия частиц в первые 10 часов контакта основан на электростатическом взаимодействии НЧ с поверхностью бактериальной мембраны.
Список литературы
Murray C.J., Ikuta K.S., Sharara F. et al. Global burden of bacterial antimicrobial resistance in 2019: A systematic analysis // Lancet. 2022. V. 399. P. 629–655. https://doi.org/10.1016/S0140-6736(21)02724-0
Frei A., Verderosa A.D., Elliott A.G. et al. Metals to combat antimicrobial resistance// Nature Review Chemistry. 2023. V. 7. P. 202–224. https://doi.org/10.1038/s41570-023-00463-4
Arora N., Thangavelu K., Karanikolos G.N. Bimetallic nanoparticles for antimicrobial applications // Frontiers in Chemistry. 2020. V. 8. P. 412. https://doi.org/10.3389/fchem.2020.00412
Padilla-Cruz A.L., Garza-Cervantes J.A., Vasto-Anzaldo X.G. et al. Synthesis and design of Ag−Fe bimetallic nanoparticles as antimicrobial synergistic combination therapies against clinically relevant pathogens // Scientific Reports. 2021. V. 11. № 1. P. 1–10. https://doi.org/10.1038/s41598-021-84768-8
Akter M., Sikder M.T., Rahman M.M. et al. A systematic review on silver nanoparticles-induced cytotoxicity: Physicochemical properties and perspectives // Journal of Advanced Research. 2017. V. 2. № 9. P. 1–16. https://doi.org/10.1016/j.jare.2017.10.008
Chen M., Shou Z., Jin X. et al. Emerging strategies in nanotechnology to treat respiratory tract infections: Realizing current trends for future clinical perspectives // Drug Delivery. 2022. V. 29. № 1. P. 2442–2458. https://doi.org/10.1080/10717544.2022.2089294
Ferrando R., Jellinek J., Johnston R.L. Nanoalloys: From theory to applicalions of alloy clusters and nanoparticles // Chemical Review. 2008. V. 108. № 3. P. 845‒910. https://doi.org/10.1021/cr040090g
Nasrabadi H.T., Abbasi E., Davaran S. et al. Bimetallic nanoparticles: Preparation, properties, and biomedical applications // Artificial Cells, Nanomedicine, and Biotechnology. 2016. V. 44. № 1. P. 376‒380. https://doi.org/10.3109/21691401.2014.953632
Belenov S.V., Volochaev V.A., Pryadchenko V.V. et al. Phase behavior of Pt−Cu nanoparticles with different architecture upon their thermal treatment // Nanotechnologies in Russia. 2017. V. 12. P. 147–155. https://doi.org/10.1134/S1995078017020033
Banerjee M., Sharma S., Chattopadhyay A. et al. Enhanced antibacterial activity of bimetallic gold-silver core-shell nanoparticles at low silver concentration // Nanoscale. 2011. V. 3. № 12. P. 5120‒5125. https://doi.org/10.1039/C1NR10703H
Alonso A., Vigués N., Muñoz-Berbel X. et al. Microbial growth inhibition caused by Zn/Ag‒Y zeolite materials with different amounts of silver // Colloids and Surfaces B: Biointerfaces. 2016. V. 142. P. 141‒147. https://doi.org/10.1016/j.colsurfb.2016.02.042
Ferreira L., Guedes J.F., Almeida-Aguiar C. et al. Microbial growth inhibition caused by Zn/Ag−Y zeolite materials with different amounts of silver // Colloids and Surface 2016. V. 142. P. 141‒147. https://doi.org/10.1016/j.colsurfb.2016.02.042
Markova Z., Šišková K.M., Filip J. et al. Air stable magnetic bimetallic Fe–Ag nanoparticles for advanced antimicrobial treatment and phosphorus removal // Environmental Science & Technology. 2013. V. 47. № 10. P. 5285‒5293. https://doi.org/10.1021/es304693g
Taner M., Sayar N., Yulug I.G. et al. Synthesis, characterization and antibacterial investigation of silver–copper nanoalloys // Journal of Materials Chemistry. 2011. V. 21. № 35. P. 13150‒13154. https://doi.org/10.1039/C1JM11718A
Gupta A., Mumtaz S., Li C.H. et al. Combatting antibiotic-resistant bacteria using nanomaterials // Chemical Society Reviews 2019. V. 48. № 2. P. 415–427 https://doi.org/10.1039/C7CS00748E
Rajchakit U., Sarojini V. Recent developments in antimicrobial-peptide-conjugated gold nanoparticles // Bioconjugate Chemistry. 2017. V. 15. № 28. P. 2673‒2686. https://doi.org/10.1021/acs.bioconjchem.7b00368
Букина Ю.А., Сергеева Е.А. Антибактериальные свойства и механизм бактерицидного действия наночастиц и ионов серебра // Вестник Казанского технологического университета. 2012. Т. 15. № 14. С. 170‒172.
Pillai P., Kowalczyk P.B., Kandere-Grzybowska K. et al. Engineering gram selectivity of mixed-charge gold nanoparticles by tuning the balance of surface charges // Angewandte Chemie International Edition. 2016. V. 55. № 30. P. 8610‒8614. https://doi.org/10.1002/anie.201602965
Huo S., Jiang Y., Gupta A. et al. Fully zwitterionic nanoparticle antimicrobial agents through tuning of core size and ligand structure // ACS Nano. 2016. V. 10. № 9. P. 8732‒8737. https://doi.org/10.1021/acsnano.6b04207
Krishnan G., de Graaf S., Gert H. et al. Strategies to initiate and control the nucleation behavior of bimetallic nanoparticles // Nanoscale. 2017. V. 9. № 24. P. 8149‒8156. https://doi.org/10.1039/C7NR00916J
Langlois C., Li Z.I., Yuan J. et al. Transition from core–shell to Janus chemical configuration for bimetallic nanoparticles // Nanoscale. 2012. V. 4. № 11. P. 3381‒3388. https://doi.org/10.1039/C2NR11954D
Bakina O.V., Glazkova E.A., Svarovskaya N.V. et al. “Janus”-like Cu−Fe bimetallic nanoparticles with high antibacterial activity // Materials Letters. 2019. V. 242. P. 187‒190. https://doi.org/10.1016/j.matlet.2019.01.105
Lozhkomoev A.S., Kazantsev S.O., Kondranova A.M. et al. Design of antimicrobial composite nanoparticles ZnxMe (100 – x)/O by electrical explosion of two wires in the oxygen-containing atmosphere // Materials & Design. 2019. V. 183. P. 108099. https://doi.org/10.1016/j.matdes.2019.108099
Bakina O.V., Glazkova E.A., Pervikov A.V. et al. Electric explosion of wires as versatile method for antibacterial Janus-like ZnO–Ag nanoparticles preparation // J. Mater. Sci. Materials in Electronics. 2021. V. 32. P. 10623‒10634. https://doi.org/10.1007/s10854-019-01684-4
Bakina O., Glazkova E., Pervikov A. et al. Design and preparation of silver–copper nanoalloys for antibacterial applications // Journal of Cluster Science. 2021. V. 32. P. 779‒786. https://doi.org/10.1007/s10876-020-01844-1
Chace W.G. Exploding wires // Phisics Today. 1964. V. 17. № 8. P. 19. https://doi.org/10.1063/1.3051737
Кузнецова А.С., Ермакова Л.Э., Антропова Т.В. и др. Химический состав, структура и электрокинетический потенциал никель- и железосодержащих стеклообразных материалов // Коллоидный журнал. 2021. Т. 83. № 3. С. 311‒319. https://doi.org/10.31857/S0023291221030101
Balouiri M., Sadiki M., Ibnsouda S.K. Methods for in vitro evaluating antimicrobial activity: A review // Journal of Pharmaceutical Analysis. 2016. V. 6. № 2. P. 71‒79. https://doi.org/10.1016/j.jpha.2015.11.005
Lerner M.I., Svarovskaya N.V., Psakhie S.G. et al. Production technology, characteristics, and some applications of electric-explosion nanopowders of metals // Na-notechnologies in Russia. 2009. V. 4. № 11–12. P. 741–757. https://doi.org/10.1088/09574484/27/20/205603
Диаграммы состояния двойных металлических систем / под общей редакцией Лякишева. Т. 1. М.: Машиностроение, 1996. 996 с.
Чудненко К.В., Пальянова Г.А. Термодинамические свойства твердых растворов в системе Ag–Au–Сu // Геология и геофизика. 2014. Т. 55. № 3. С. 449‒463.
Liu X., Wu Y., Xie G. et al. New green soft chemistry route to Ag−Cu bimetallic nanomaterials // International Journal of Electrochemical Science. 2017. V. 12. P. 3275‒3282. https://doi.org/10.20964/2017.04.61
Williamson G.K., Hall W.H. X-ray line broadening from filed aluminium and wolfram // Acta Metallurgica. 1953. V. 1. P. 22‒31. https://doi.org/10.1016/0001-6160(53)90006-6
Kushwah M., Gaur M.S., Berlina A.N. et al. Biosynthesis of novel Ag@ Cu alloy NPs for enhancement of methylene blue photocatalytic activity and antibacterial activity // Materials Research Express. 2019. V. 6. № 11. P. 116561. https://doi.org/10.1088/2053-1591/ab485e
Ni Z., Wan M., Tang G. et al. Synthesis of CuO and PAA-regulated silver-carried CuO nanosheet composites and their antibacterial properties // Polymers. 2022. V. 14. № 24. P. 5422. https://doi.org/10.3390/polym14245422
Kaushik V.K. XPS core level spectra and Auger parameters for some silver compounds // Journal of Electron Spectroscopy and Related Phenomena. 1991. V. 56. № 3. P. 273‒277. https://doi.org/10.1016/0368-2048(91)85008-H
Rajendran R., Mani A. Photocatalytic, antibacterial and anticancer activity of silver-doped zinc oxide nanoparticles // Journal of Saudi Chemical Society. 2020. V. 24. № 12. P. 1010‒1024. https://doi.org/10.1016/j.jscs.2020.10.008
Parvin T., Keerthiraj N., Ibrahim I.A. et al. Photocatalytic degradation of municipal wastewater and brilliant blue dye using hydrothermally synthesized surface-modified silver-doped ZnO designer particles // International Journal of Photoenergy. 2012. V. 2012. P. 670610. https://doi.org/10.1155/2012/670610
Chan Y.Y., Pang Y.L., Lim S. et al. Biosynthesized Fe-and Ag-doped ZnO nanoparticles using aqueous extract of Clitoria ternatea Linn for enhancement of sonocatalytic degradation of Congo red // Environmental Science and Pollution Research. 2020. V. 27. P. 34675‒34691. https://doi.org/10.1007/s11356-019-06583-z
Azouri A., Ge, M., Xun K. et al. Zeta potential studies of titanium dioxide and silver nanoparticle composites in water-based colloidal suspension // Multifunctional Nanocomposites and Nanomaterials International Conference. 2008. V. 47616. P. 221–223. https://doi.org/10.1115/MN2006-17072
Ren Y., Wang C., Chen Z. et al. Emergent heterogeneous microenvironments in biofilms: substratum surface heterogeneity and bacterial adhesion force-sensing // FEMS Microbiology Reviews. 2018. V. 42. № 3. P. 259‒272. https://doi.org/10.1093/femsre/fuy001
Дополнительные материалы отсутствуют.
Инструменты
Коллоидный журнал